Terahertz measuring instruments are entering a phase of industrial application and now represent a significant potential market.
ANSELM DENINGER, LISA GRAY and TIM PAASCH-COLBERG, TOPTICA Photonics
Just a few years ago, the application of terahertz radiation seemed obscure at best. If experts were asked for killer applications, few could be named. In 2018, however, terahertz measuring instruments are showing significant market potential. Applications in the field of civil safety, nondestructive testing, and industrial quality control all profit from a new generation of terahertz systems. Depending on the actual applications, several different types of technologies have merit.
Photomixers with fiber pigtails. The active structure sits at the center of the cylindrical package; the generated terahertz radiation is emitted through the silicon lens.
The terahertz spectrum contains frequencies from 100 GHz to 10 THz (wavelengths between 3 mm and 30 µm) and fills a gap between IR light and microwaves (Figure 1). The generation of terahertz radiation can be achieved using a multitude of techniques. The applications described below employ optoelectronic sources that rely on the conversion of NIR laser light to terahertz waves. Compared to alternative techniques — for example, frequency multipliers or quantum cascade lasers — optoelectronic systems are characterized by increased robustness, more compact footprints, and very broad, usable bandwidth.
Figure 1. The terahertz range refers to electromagnetic waves between IR light and microwaves.
There are three emerging applications, each with differing systems and instrumentation: (1) the sensitive detection of trace gases, which calls for systems with high spectral resolution and where frequency-domain spectrometers appear best suited; (2) layer thickness measurements, which benefit from time-domain systems, as in the extrusion of plastic pieces and the characterization of paint layers in the automobile industry; and (3) real-time observation of samples on fast-moving conveyor belts, demonstrated with a rapid screening system that measures up to 500 kilosamples per second. In the interim, one can expect that increased market acceptance of these systems will lead to greater volume effects in the production of components.
Gas detection
Frequency-domain spectroscopy uses the principle of difference-frequency mixing of two tunable lasers. The light of two slightly different wavelengths illuminates a specific semiconductor component, or photomixer (opening image), which converts the beat signal of the wavelengths into terahertz radiation. The wavelengths of diode lasers, in particular, can be precisely controlled, so that the resulting terahertz radiation is also “on point” and can be set or scanned easily. A frequency resolution of just 1 MHz pays off in trace gas analysis; several gases possess distinct transitions in the terahertz frequency range, which narrow down at low pressure and can then be identified by their absorption fingerprint.
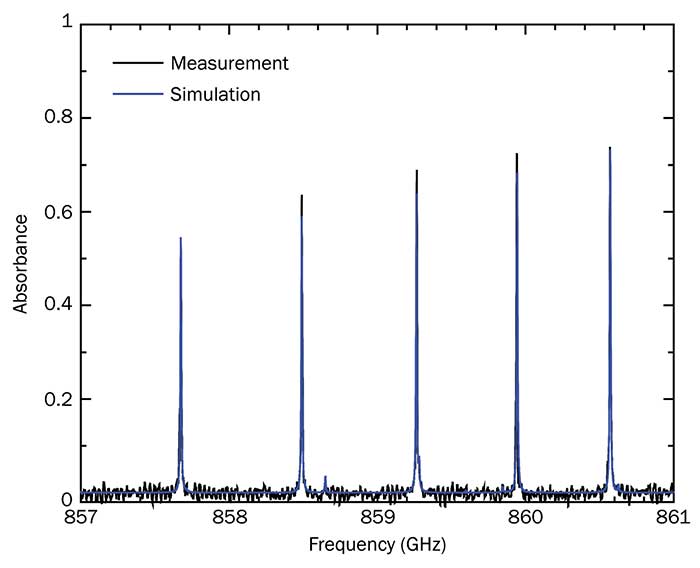
Figure 2. Close-up of the terahertz absorption spectrum of sulfur dioxide: The TeraScan resolves line profiles with a width of only a few megahertz. Experimental data (black line) and literature values (blue line) are in excellent agreement.
Germany’s Federal Ministry for Education and Research funded a project between 2014 and 2017 that looked at the precise detection of poisonous gases in industry buildings. The project consortium included TOPTICA Photonics, the Fraunhofer Heinrich Hertz Institute in Berlin, the Analytical Task Force of the fire department of Mannheim, Germany, and other partners. They conceived a mobile measuring station based on a high-precision frequency domain spectrometer and analyzed scenarios that included the protection of production lines from explosion and the clearing of a dangerous situation in case of emergency. In both cases, it was necessary to gather precise information about the type and amount of poisonous gases released. The measuring station reached a detection limit of approximately 10 ppm with ammonia, and 100 ppm with hydrogen sulfide and sulfur dioxide. Figure 2 shows a representative absorption spectrum of sulfur dioxide at low pressure.
Layer thickness measurements
Terahertz measurements in the time domain are based on pulsed sources. In contrast to frequency-domain spectroscopy, these systems use only one laser that emits short IR pulses with a duration of 50 to 100 fs. The laser pulses hit a photoconductive switch and create short current transients, which contain high (terahertz) frequency components. The best photoconductive switches currently available make use of indium gallium arsenide (InGaAs) semiconductor material and achieve bandwidths up to 7 THz.
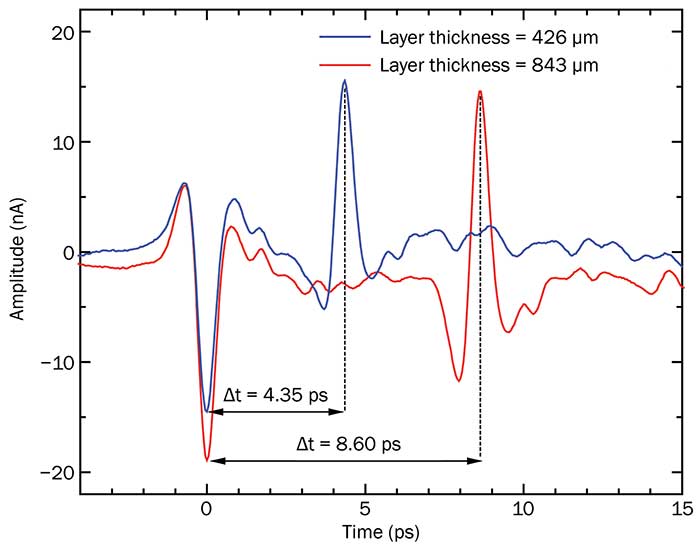
Figure 3. Terahertz pulses reflected off different spots of a plastic bottle. In the same bottle, the wall thickness varies by a factor of almost two. Terahertz echolocation easily detects these inhomogeneities.
In the quality control of plastic pieces or the inspection of paint and coating layers, one of the most promising industrial applications — the measurement of thin, optically opaque layers — benefits from short pulses. The measuring principle is similar to echolocation. Terahertz pulses are focused on the layer under inspection, and the top and bottom side each reflect a part of the incident pulse. If the refractive index of the material is known, we can calculate the thickness of the layer based on the time-of-arrival of the pulses (Figure 3). This method even works with multilayered surfaces, as long as the individual materials have a different refractive index. The time-domain system TeraFlash has resolved layers of 10- to 20-µm thickness.
Screening for quality assurance
A third group of industrial applications requires neither spectral measurements nor thickness information but an extremely fast recording of intensity values. Rapid recording is nicely illustrated with this quality-control scenario. According to a directive from the EU Parliament, medications may only be distributed to patients if a delivery note with patient information is included. Production facilities must insert the delivery note into the packaging before inserting the medication. (Delivery notes are made of papers or plastics, and the folded medication boxes are made of cardboard.) Once delivery notes and medications have been inserted, the entire weight is tallied. However, should the sums not match, it is impossible for quality control to locate the individual packages without delivery notes. The speed of the conveyor belts — often greater than 10 m/s — is too fast for in-line measurements.
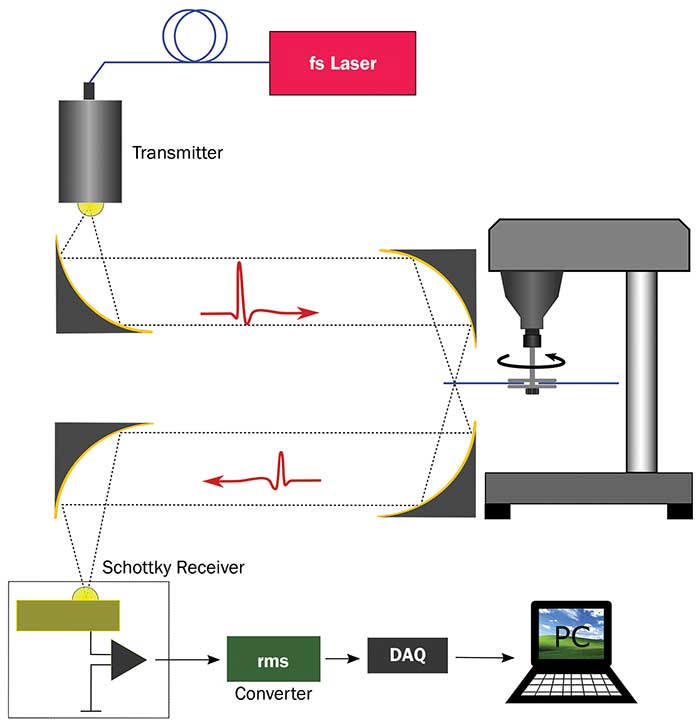
Figure 4. Terahertz setup to analyze folded cardboard boxes. The transmitter converts the output of a femtosecond (fs) laser to terahertz pulses. Parabolic mirrors focus the pulses onto the samples mounted on a fast turntable. The Schottky receiver measures the transmitted intensities, which are further processed in a root mean square (rms) converter and a data acquisition (DAQ) unit.
In a feasibility experiment conducted by TOPTICA Photonics, folded boxes were mounted on an industrial drill that simulated a fast conveyor belt. The boxes rotated with a transversal speed of 21 m/s through the focus of the terahertz beam. The dips in the time-dependent signal result from the scattering of the terahertz pulses at the edges of the sample itself and in the paper fold. The presence of a delivery note becomes visible in two additional peaks in the signal. The experiments show that packages with missing delivery notes can still be identified. The method even worked for samples that overlapped in a tile-like manner, with an overlap degree up to 50 to 60 percent (Figures 4, 5).
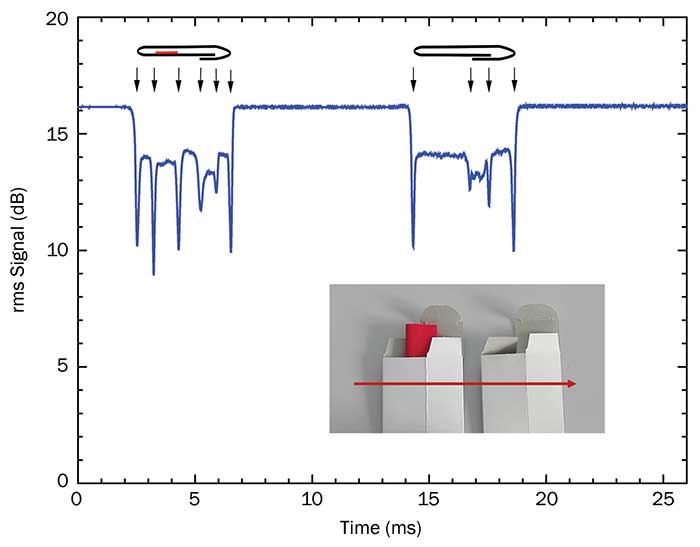
Figure 5. Terahertz transmission through folded cardboard boxes with (left) and without (right) a delivery note. In this measurement, the boxes moved at 21 m/s; rms: root mean square.
New procedures can measure the intensity of each and every terahertz pulse. The measurement process can be implemented in a screening system and enable an acquisition speed of 100 million data points per second. Because such large data volumes are not easily processed, it is advantageous to average several thousand measuring points at a time. Even then, the data rate is still considerably higher than earlier terahertz systems and sufficient to deliver a high resolution at even sample speeds well beyond 100 km/h.
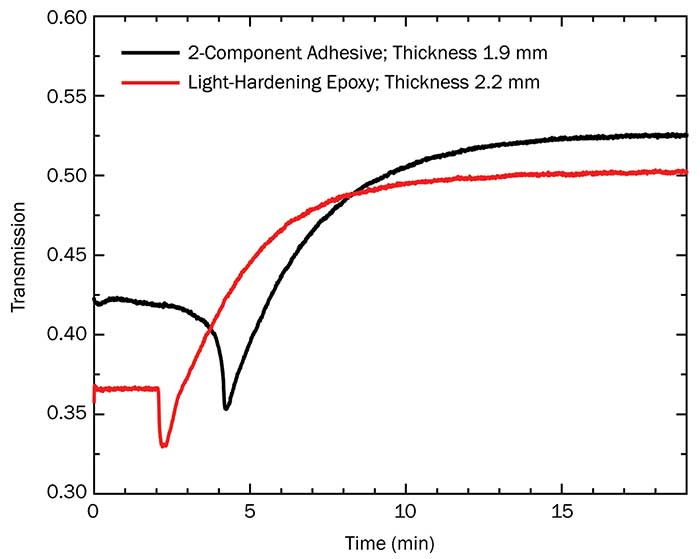
Figure 6. Temporal evolution of the curing process of a transparent two-component adhesive (black curve) and a light-hardening epoxy adhesive (red curve), recorded with a TeraSpeed.
Unlike conventional time-domain spectrometers, more recent screening systems do not use any moving parts and are thus extremely robust, both thermally and mechanically. The data rate can be reduced if slow processes, such as the curing of adhesives (Figure 6), have to be observed. Initial research along these lines has shown that the transmission properties of a two-component adhesive and a light-hardening epoxy adhesive are significantly changed in the curing process. Terahertz measurements thus allow a touch-free control of the tempering procedure and can therefore help to optimize the curing duration and material composition.
The unique characteristics of terahertz radiation turn it into an effective tool for a variety of applications.
Looking to the future, and beyond the applications mentioned here, higher carrier frequencies will enable higher data rates in wireless communication. And there has been recent work characterizing pharmaceuticals, both with respect to their structure (coating thickness) and composition. The number of emerging applications — especially in contact-free material and quality testing — is constantly growing.
Meet the authors
Anselm Deninger, Ph.D., is product manager and director of terahertz technology at TOPTICA Photonics AG. He received a doctorate in physics from the University of Mainz, having investigated the applications of spin-polarized helium-3 gas to functional magnetic resonance imaging of human lungs; email: [email protected].
Lisa Gray currently holds an inside sales and marketing position at TOPTICA Photonics. She has a bachelor’s degree in international relations with a minor in business administration from SUNY Geneseo; email: [email protected].
Tim Paasch-Colberg, Ph.D., was marketing director at TOPTICA Photonics AG and responsible for worldwide marketing of
TOPTICA’s product lines. His doctorate in physics from the University of Munich focused on charge carrier dynamics in solid-state materials on attosecond timescales; email: [email protected].