A new way has been discovered to build visible-wavelength solid-state lasers, primarily for use in biotechnology applications.
Vincent Issier, Marc von Gunten, Thomas Kraft, William Minford and Andre Wong, JDSU Corp.
The telecom industry demands extreme reliability in lasers and photonic components, so very mature assembly and packaging techniques have evolved to meet these demands over the years. Integration of these techniques from the telecom industry has now allowed us to design low-power, visible-wavelength solid-state lasers. To our knowledge, this is the first instance of telecom packaging technology’s being integrated into commercial visible lasers.
The new design is driven by the market for low-power continuous-wave visible lasers. The dominant application for these lasers is biotechnology instruments that use laser-induced fluorescence and light-scattering techniques. Smaller market segments exist in graphics and in imaging and semiconductor front-end instrument applications.
In previous decades, these applications relied on such gas-discharge lasers as argon-ion and helium-neon. However, the lifetime limitations of these lasers, together with their package size, electrical efficiency and heat-load considerations, have led to new instrument designs that use solid-state lasers instead (instruments using gas lasers have not completely disappeared yet).
The familiar 532-nm solid-state lasers have been commercially available from numerous manufacturers for more than a decade. More recently, the many existing applications for the 488-nm wavelength of the old argon-ion lasers have led to the emergence of commercial solid-state lasers at that wavelength.
These diode-pumped solid-state (DPSS) lasers already have dramatically improved the efficiency and lifetime and reduced the package size compared with the old gas-discharge lasers. Nonetheless, the market demands further improvements in these parameters, often combined with fiber output coupling, as users seek easy-to-use desktop instruments with ever more features.
At the same time, consistent high-volume manufacturing must be guaranteed because, in coming years, the market will grow to more than 25,000 lasers annually — much larger than the market for gas-discharge lasers ever was. This is because solid-state lasers allow for easier instrument design and integration and provide wavelengths and powers that have not been available with gas-discharge lasers.
These considerations have led us to re-examine the conventional approach to designing DPSS lasers — a method in which the pump laser, laser crystal, mirrors, lenses and nonlinear crystals are mounted on an optical base plate, which in turn is mounted on a relatively large thermoelectric cooler.
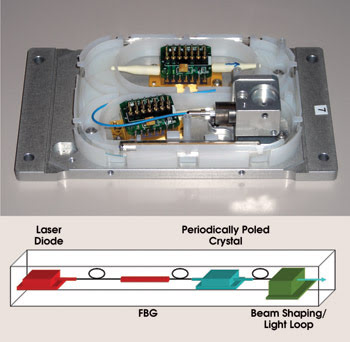
Figure 1. The schematic diagram shows the key laser components, which are folded inside the package shown in the photograph.
Our alternative invokes telecom technology in a simpler fiber-based design (Figure 1). We house a 976-nm single-mode laser diode in a standard butterfly package, with a fiber Bragg grating (FBG) stabilizing the laser’s wavelength and serving as its output coupler. The emerging 976-nm power is coupled with a single-mode polarization-maintaining fiber into a periodically poled lithium niobate waveguide, where its frequency is doubled to 488 nm. The waveguide also is in a butterfly package.
The resulting blue light is collected into another single-mode polarization-maintaining fiber and transported to the final beam-shaping optics (not shown in the photograph in Figure 1). A feedback loop at the output stabilizes the power level. Both the laser diode and the periodically poled lithium niobate waveguide are temperature-stabilized by small thermoelectric coolers integrated into their butterfly packages.
This fiber-based telecom-style architecture delivers excellent performance. The mode quality is very close to M2 = 1, as expected, because the light is emitted from a single-mode fiber. The beam-pointing stability is extremely good because, in a fiber-coupled laser, it is defined by the mounting of the fiber and not, as in a standard DPSS laser, by the mechanical stability of the laser cavity. The fiber-coupled design also allows relatively fast warm-up times and power level changes because the thermoelectric coolers must stabilize the temperature of only very small volumes — the laser diode chip and the periodically poled lithium niobate waveguide — and not a whole DPSS baseplate. Typical warm-up behavior is shown in Figure 2.
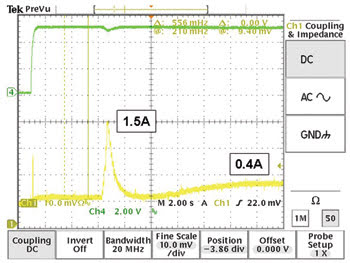
Figure 2. The upper oscilloscope trace is the DC voltage, and the lower one is the current. The laser warms up in about 2 s with a maximum current of 1.5 A, then settles down to an operating current of 0.4 A, or 2 W of electrical power.
The new design is significantly more efficient than conventional designs. For one thing, the smaller thermoelectric coolers draw less current, and, probably more importantly, the efficiency improvement recently achieved in laser diodes translates directly into enhanced efficiency — and less heat loading — for the entire system. Figure 3 compares the heat loading for the telecom-based design with that for a conventional DPSS laser.
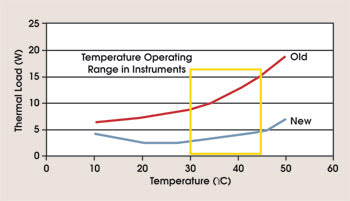
Figure 3. The telecom-based design dissipates roughly one-third the heat of conventional DPSS designs. Typical instrument operating temperature ranges are indicated by yellow box.
The lower operating currents for the thermoelectric coolers and the laser diode also enable a higher package density of the electronic components on the controller board. This allows integration of the control electronics with the optics in one common housing. In Figure 1, the electronic board has been removed to reveal the optical components beneath it, but in the packaged laser, the electronics sit directly above the optical components.
The improved electrical efficiency of the telecom-based design solves two instrument design issues. First, it produces a smaller, more compact instrument thanks to integrated control electronics that need no heat sink and only a small DC power supply. This corresponds to a volume reduction of about 80 percent compared with standard DPSS laser designs.
Secondly, the electrical efficiency reduces the beam-pointing issues related to heating of the optical platform, which can lead to mechanical distortion that deflects the beam.
The fiber-based telecom-style architecture enables a true fiber delivery option with the same performance parameters as a free-space version. The optomechanical stability of a pigtailed butterfly package is superior to that of a conventional DPSS coupled into a fiber.
Manufacturability is a very important consideration for any laser with a large market volume. Standard DPSS lasers, which require the alignment of up to 10 optical components on a baseplate, can be challenging, especially in single-longitudinal-mode cavities.
The telecom-based design, however, requires far fewer mechanical alignments. Automatic alignment stations, similar to the ones used for telecom components, can be used to package the fiber-pigtailed laser diode and waveguide. As such, the requirements to manufacture a laser fit nicely into existing manufacturing processes that are used for high-volume manufacturing of telecom components. Manufacturing the laser comes down to splicing together fiber-pigtailed components — no complicated alignments of a relatively large number of optical components to a baseplate are necessary.
The design discussed here has been fully implemented in a commercial product, the FCD488 laser. We believe that the laser brings the maturity and reliability of telecom packaging to the continuous-wave visible laser market.
Meet the authors
Vincent Issier is a program manager at JDSU Commercial Lasers Products Group, JDSU Corp., in Milpitas, Calif.; e-mail: [email protected].
Marc von Gunten is a program manager, JDSU Advanced Optical Technologies Products Group; e-mail: [email protected].
Thomas Kraft is a product line manager, JDSU Commercial Lasers Products Group; e-mail: [email protected].
William Minford is a program manager, JDSU Optical Communications Products Group; e-mail: [email protected].
Andre Wong is a project manager, JDSU Optical Communications Products Group; e-mail: [email protected].