A German research team has completed the preliminary design for an ultracompact quantum communication payload that sends entangled photons to two ground stations simultaneously.
ERIK BECKERT AND DANIEL HEINIG, FRAUNHOFER IOF, AND ANDREAS THOSS, CONTRIBUTING EDITOR
Entanglement quantum key distribution (QKD) is a fundamental principle for securing future communication networks. For this type of QKD, entangled photons are generated and sent to two separate receivers, often referred to as “Alice and Bob.” These photons cannot be intercepted without disrupting their entangled states. And, because Alice and Bob are also connected by a conventional network, they can openly share information about the quality of entanglement that each photon shares, and thus convert a sequence of entangled photons into a secret key. Afterward, they can exchange data encrypted with this quantum key via conventional connections. Importantly, unlike other types of QKD, Alice and Bob do not need to trust the source of their entangled photons.
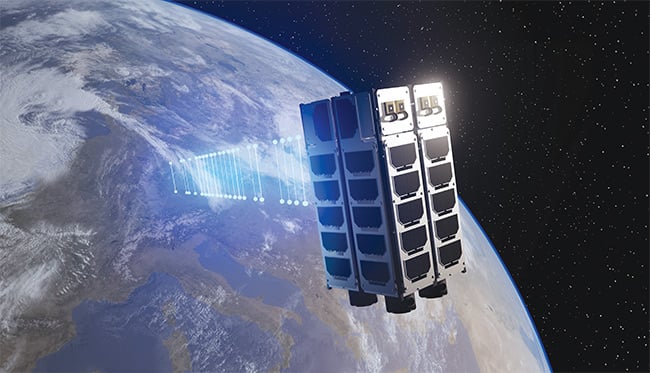
A team led by researchers at the Fraunhofer Institute for Applied Optics and Precision Engineering (Fraunhofer IOF) undertook the challenge of building a CubeSat-based quantum key distribution (QKD) system. The preliminary design illustrated how entanglement can be distributed from a space-based platform to two ground stations. Courtesy of Fraunhofer IOF.
Entanglement QKD systems can be established via fiber connections for landlines. Implementation is more challenging for satellite-based communication, although QKD offers substantial benefits to space-based networks. In space, for example, they can transmit quantum keys over much longer distances than via fibers. The reason for this lies in the nature of entangled photons themselves: They cannot be amplified. Amplification destroys the unique quantum character of these photons, and therefore QKD in optical fibers is limited to the distance that a photon can travel without amplification in a fiber.
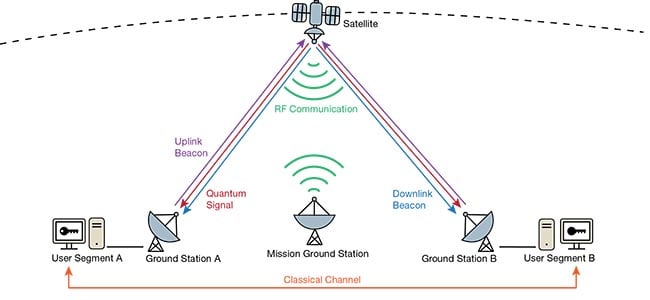
Figure 1. The general scope of the CubEniK satellite mission aims to achieve distribution of entangled photons from a satellite to two separate ground stations. Courtesy of Fraunhofer IOF.
Typically, this distance is <200 km. In space-based entanglement QKD, a satellite sends entangled photons to the Alice and Bob ground stations through free space. Depending on the satellite’s orbit, Alice and Bob can be >1000 km apart.
The project
In 2016, China-based researchers comprised the first team to launch a satellite fielding two QKD experiments. Within each experiment they focused on a different QKD technique.
In one approach in 2017, the researchers established a video link between teams in Beijing and Vienna, Austria, by means of a technique called prepare-and-measure QKD. They sent one sequence of photons to the Chinese ground station first, stored the signal on the satellite, and sent a second sequence to the team in Austria, which allowed both locations to create a quantum key for secure communication between them. However, this procedure is not entirely safe, because storing the sequence on the satellite allows eavesdropping to occur on the satellite.
More recently, a team of researchers in Germany led by the Fraunhofer Institute for Applied Optics and Precision Engineering (Fraunhofer IOF) in Jena took on the challenge of building its own satellite-based entanglement QKD system, and they did so with the further aim of packaging it in the smallest form factor possible: a CubeSat.
Funded by the German Federal
Ministry for Economic Affairs and
Climate Action, the team completed
the preliminary design phase for what they dubbed the CubEniK project. The acronym stands for “CubeSat Demonstration of Entangled Photon Pair Key Distribution.”
The CubEniK consortium unites the Fraunhofer spinoffs Quantum Optics Jena and SPACEOPTIX. Each respectively provides the mission design and telescope optics. They are complemented by the Center for Telematics in Würzburg, which performs the demonstrator tests, and DiGOS GmbH, which offers experience with laser ranging, ground stations, and ground station operators.
The team from Fraunhofer further brings experience with engineering optical and electronic systems for space applications, and space-grade, high-performing entangled photon sources that deliver repetition rates in the gigahertz range. Two ground stations for the project are based in Germany: one in Jena and
the other at the DLR Institute of Communications facility in Oberpfaffenhofen.
The project aims to simultaneously provide a secure key with a length of 256 bits to each ground station using the BBM92 distribution protocol within one overflight of the satellite. This key length could serve as a master key in high-security modules for the financial industry or governmental authorities.
The optomechanical design
Even though satellites have recently become another mass-produced product, every gram of weight still counts. This
is why CubeSats have become popular:
As a class of nano- or microsatellites, they offer a minimal footprint that
allows them to be carried as a secondary payload on larger launch missions.
CubeSats were proposed by Stanford University professors in 1999 to make satellite platforms more accessible to
their graduate students’ research. These space platforms are designed to provide cubes of 10 cubic cm (or 1 L) of useful volume in a satellite. Typical configurations contain six or 12 of these boxes and are therefore called 6U or 12U configurations. Larger platforms such as 16U are much rarer, and so are their launch opportunities.
In the beginning, the CubEniK consortium aspired to put the whole payload
into a 6U to 10U pack. It turned out,
however, that the two telescopes that
were needed to address two ground
stations did not simultaneously fit into this space. The plan had to be changed
to develop a 16U configuration, which posed severe consequences for launch opportunities.
Nevertheless, the optical payload of the CubEniK is still the smallest system of its kind to be published. The size of the telescopes in commercial laser terminals can be smaller because they do not need to handle single photons. Figure 2 shows the preliminary design where the optical payload fits into 8U.
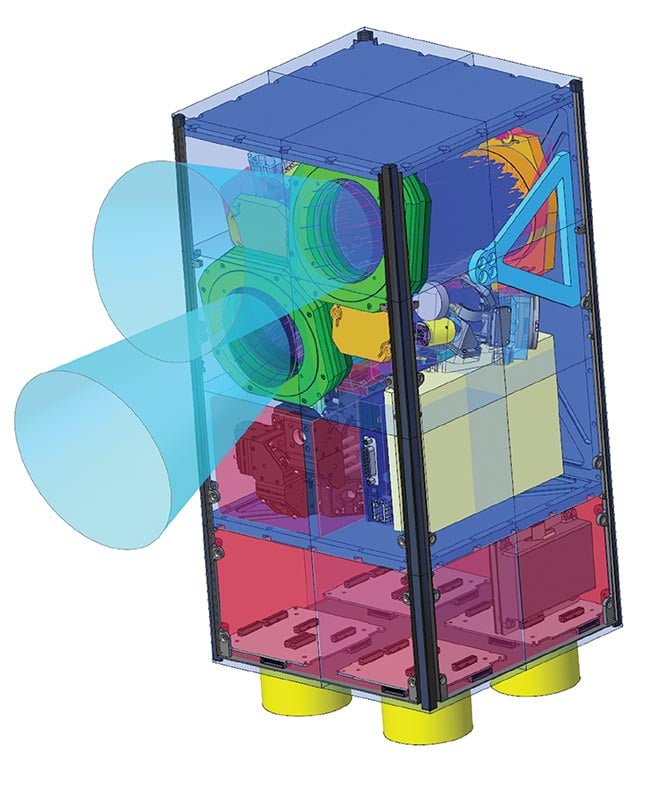
Figure 2. CubeSat platforms are designed to apportion 10-cubic-cm cubes of useful volume in a satellite. A platform configured with eight such cubes is termed an 8U configuration. The preliminary payload design for Fraunhofer IOF’s CubeSat is a 16U configuration. The optical payload occupies the top 8U portion, while the electronic payload, including the photon source, fills the mid 4U, and the satellite platform the lower 4U. Courtesy of Fraunhofer IOF.
The telescopes within the new setup
are based on off-the-shelf technology from project partner SPACEOPTIX.
Within the envelope of the telescopes, the engineers from Fraunhofer IOF designed an additional space (blue box in Figure
3) which comprises the fine pointing
assembly (FPA) and the fiber coupler.
The FPA and the subsequent coarse
pointing assembly (CPA) (green units in Figure 3) stabilize the beam as it is
directed toward the ground stations. Stabilization is performed by two components. The first is a piezo-driven tip-tilt mirror (gray disk on the blue box in Figure 3) for microrad steering. The second relies on a set of two wedge prisms in the CPA. The latter form a so-called Risley prism scanner, in which independently rotatable prisms allow the beam to be tilted to up to 1°. This solution proved to be more compact than gimbals.
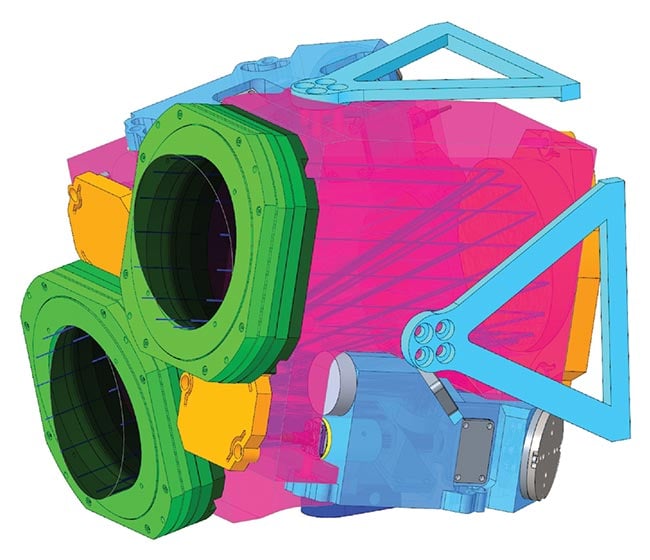
Figure 3. The optical payload of the CubEniK CubeSat contains two telescopes (pink), as well as a coarse pointing assembly (CPA) (green), beam combination components (blue), and a fine pointing assembly. Courtesy of Fraunhofer IOF.
The fine pointing function must be precise — in the range of a few microrads — to transmit sufficient data. The CPA allows tracking of the ground stations during flyover. The telescopes have an aperture of 80 mm with outer dimensions
of 150 × 100 × 177 mm. The larger aperture results in smaller divergence of the signal beam, which also increases the transmission rate of the single photon quantum signals.
Two such telescopes are fixed against each other at an angle chosen to help minimize the thickness of the Risley prisms. Making the prisms as thin as
possible without contributing much
dispersion on the quantum signals was one of the major challenges the optical designers confronted. One telescope
receives the uplink beacon and sends it to a position sensor that enables control of the steering angle.
The blue box in Figure 3 contains two fiber ports, one for the quantum signal and one for the beacon laser. Though fiber
coupling is sometimes difficult in space applications, it proved to be the optimal solution for the volume-optimized setup in the payload.
The photon source must generate at least 1 million polarization-entangled pairs per second so that the 256-bit key can be transmitted through the attenuating atmosphere to the ground stations, Alice and Bob, during each flyover.
The team at Fraunhofer IOF, which has
designed and built such sources for more than a decade, designed a palm-sized module for the CubeSat mission. The module comprises a wavelength-stabilized laser and a Sagnac interferometer. They chose a wavelength centered ~800 nm, though 1550-nm sources are also available. The source and the telescope are connected by polarization-maintaining fibers.
Searching for a new launch platform
The CubEniK consortium has completed the preliminary design for an ultracompact payload for a satellite mission for simultaneous distribution of entangled photons to two separate ground stations. The design fits into a 16U CubeSat and shall allow for the distribution of a 256-bit quantum key during one flyover in a low Earth orbit. The design is the smallest one ever published for such a task.
The scope of the mission places significantly greater requirements on the position control, as well as thermal and energy management compared to regular CubeSat missions. This led to the extension of the payload beyond the originally planned CubeSat dimensions. This points to a potential market demand for larger CubeSat missions. So far, such missions are limited to 12U, but it is obvious that slightly larger configurations of 16U offer extended opportunities. That might present a market for upcoming micro-launchers at the same time that they are currently evolving. Now, the project team is reconfiguring to adapt its plans to the higher payload weight. In particular, a platform for the larger weight is needed.
Meet the authors
Erik Beckert earned his diploma in precision engineering and a doctorate in optoelectronics system integration from Technical University
of Ilmenau, Germany. He now heads the Opto-mechatronical Components and Systems department at Fraunhofer IOF in Jena, Germany; email: [email protected].
Daniel Heinig is a scientific researcher at Fraunhofer IOF, where he supervises various projects in the field of adaptive optics for free-space laser and quantum communication systems; email: [email protected].
Andreas Thoss, Ph.D., is a laser physicist, founder of THOSS Media, and a contributing editor to Photonics Spectra. He has been
writing and editing technical texts, with a
focus on the field of photonics, for two
decades; email: [email protected].