Like LEDs on steroids, these optical sources bridge the gap between laser diodes and LEDs.
Christian Vélez, Lorenzo Occhi and Dr. Micaëla B. Raschle, Exalos AG
Superluminescent LEDs, also known as high-power LEDs and “laser diodes operating below threshold,” are semiconductor light sources that have a broadband output optical spectrum, like LEDs, at high power levels, like laser diodes. This combination is valuable in a variety of applications, and as a consequence, these emitters are finding commercial use.
Superluminescent LEDs are based on a PN junction embedded in an optical waveguide. When electrically biased in the forward direction, they show optical gain and generate amplified spontaneous emission over a wide range of wavelengths. They are designed to have high single-pass amplification for the spontaneous emission generated along the waveguide, but unlike laser diodes, they have insufficient feedback to achieve lasing action.
The primary technological challenge in the development of such sources is achieving high optical output powers and a smooth spectrum. The suppression of the cavity modes typically is achieved by tilting the waveguide with respect to the end facets and by applying an antireflection coating to the facets (Figure 1). With the suppression of the cavity modes, a low ripple or smooth spectrum is obtained.
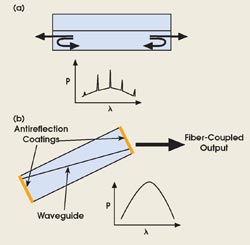
Figure 1. Reflection at the facets of a multimode Fabry-Perot laser causes wavelength resonances in the optical emission spectrum (a). Tilting the active waveguide and applying an antireflection coating on the facets suppresses the cavity resonances in a superluminescent LED, resulting in a smooth output spectrum (b).
In other words, a superluminescent LED combines the spatial coherence of a laser diode and the temporal incoherence of an LED. The spatial coherence translates into a small beam divergence, which enables the coupling of the output into a single-mode fiber with an efficiency similar to that of laser diodes. Typically more than 50 percent of the power from a single facet can be coupled into a single-mode fiber. The low temporal coherence is advantageous for applications where interferences cause problems such as speckle or ghost signals.
Superluminescent LEDs are much more powerful than standard LEDs and are particularly useful for applications that require high power densities. When biased with several hundreds of milliamperes, they typically have single-mode output powers of the same order of magnitude as single-mode laser diodes of several tens of milliwatts.
Although superluminescent LEDs have been discussed in the scientific literature for many years, they have just begun finding practical or commercial applications. Devices with high output powers, smooth spectra and large bandwidths have only recently been demonstrated and made available in large quantities.
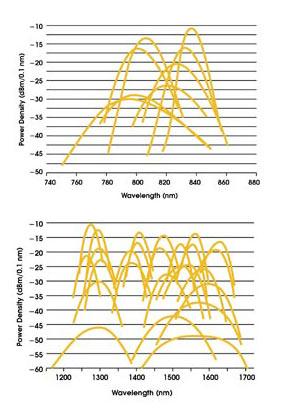
Figure 2. Stock GaAs-and InP-based superluminescent LEDs, top and bottom, respectively, are available in a variety of wavelengths.
The availability of a wide optical spectral bandwidth at high power levels makes superluminescent LEDs a cost-effective solution for a variety of purposes. The most popular wavelengths are 800, 1300 and 1550 nm, but others are commercially available, offering potential users wide flexibility (Figure 2). The LEDs operating around 800 nm are used in fiber optic gyroscopes and in medical applications, and those around 1300 and 1550 nm find most applications in telecommunications.
Operating and testing superluminescent LEDs requires the same widely available equipment as is needed to operate and test laser diodes. The similarity of these types of emitters is reflected further in their packaging. Many applications require devices with a single-mode fiber output. Because their packaging and fiber pigtailing processes are close to those known in the semiconductor laser industry, superluminescent LEDs are available in all the known laser packages.
Wide application
Optical coherence tomography (OCT) is an emerging biomedical technology that produces high-resolution cross-sectional images. Micron-scale tissue structures can be imaged in real time using the technique. The principle is analogous to that of ultrasound imaging, but OCT uses broadband light instead of sound (Figure 3).
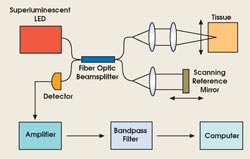
Figure 3. Optical coherence tomography uses light to generate high-resolution images of tissues.
Because light at visible and infrared wavelengths penetrates only a few millimeters into most tissue, the approach has been limited to the study of optically transparent tissues, such as those in the eye, or to endoscopic examinations.
The properties of superluminescent LEDs promise to improve the performance of OCT, dramatically changing the way that physicians, researchers and scientists see and understand the human body — and thereby empowering them to better diagnose and treat diseases.
The optical bandwidth and suppression of the cavity modes of superluminescent LEDs enable coherence lengths of tens of microns down to a few microns. The high power levels support fast scan times for ophthalmologic, cardiovascular and gastrointestinal imaging. The output powers and Gaussian beam profile enable high-resolution imaging and tissue characterization on a scale never before possible.
Another important application of superluminescent LEDs is in the navigation systems that employ fiber optic gyroscopes, which primarily are used in avionics and aerospace. At the heart of precision rotation measurement systems, fiber optic gyroscopes are based on the determination of the Sagnac phase shift of radiation propagating along a fiber optic coil when it is rotated around the winding axis. The basic elements are a light source, a single-mode polarization-maintaining fiber coil, a coupler and a detector (Figure 4).
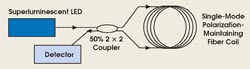
Figure 4. The fiber optic gyroscope detects rotation by measuring phase shift induced in the radiation propagating around the fiber coil.
By means of the optical coupler, light emitted by the source is injected into the fiber so that it travels in both directions around the coil. When the fiber coil is at rest, the lightwaves interfere constructively at the detector, and a maximum signal is detected. During coil rotation, the two optical waves experience different optical paths that depend on the rotation rate, and the phase difference between the two fields causes variations in the intensity detected by the photodiode, which provides information on the rotation rate. Superluminescent LED-based gyroscopes rely on the large bandwidth of the source to reduce the undesired effects of scattering along the fiber and of reflections at the facets of the internal optical components that could decrease the sensitivity for very low rotation rates.
The requirements of the optical source in a fiber optic gyroscope are low cost, small size, reliability, stability and low power consumption. Long-term wavelength stability is a demand that broadband sources, including superluminescent LEDs, have had difficulty satisfying. Consequently, fiber-based sources such as erbium-doped fiber amplifiers have tended to find application in fiber optic gyroscopes.
Exalos AG of Zurich, Switzerland, has overcome this drawback with the development of superluminescent LEDs that are less prone to wavelength shift induced by temperature or aging. Novel materials and structures have made it possible to improve the wavelength stability of the emitters by a factor of four compared with conventional superluminescent LEDs. The work is in progress, with the target of achieving wavelength stabilities on the order of 10 parts per million. Devices with this performance will substantially help to reduce size and costs in today’s manufactured fiber optic gyroscopes.
Broadband optical sources also are used in fiber optics for the testing of components or for network management purposes. An example of the first is the characterization of optical components for coarse wavelength division multiplexing (WDM), which requires a multiwavelength source. The compact size of superluminescent LEDs enables the integration of multiple emitters into a single piece of test equipment to offer wavelengths spanning the entire coarse WDM band. In optical networks, these LEDs can be used as optical sources in the measurement of the polarization mode dispersion of optical fibers.
Superluminescent LEDs have several advantages over other sources for these telecom applications. The emitters are fully compatible with the wavelengths that are used in the optical communications systems. Their optical waveguide, as mentioned, enables the efficient coupling of their output into single-mode optical fibers. And they answer well to the pressures in today’s telecom carrier market, which requires test solutions that are cost-effective and reliable, provide better productivity and reduce the number of testers in the field.
A final example of an important application for superluminescent LEDs involves fiber optic sensors for strain and temperature measurements in civil engineering, structural analysis and composite materials manufacturing. Fiber optic sensors have a number of advantages over conventional sensors. Their immunity to electromagnetic fields, their ability to measure at many points along a single fiber, and their ability to be embedded within or bonded to structures makes them a highly flexible solution.
Over the past 25 years, these sensors have seen a continuous improvement in quality and performance. The large optical bandwidth and robustness of superluminescent LEDs make them particularly suited to this application.
Meet the authors
Christian Vélez is CEO, Lorenzo Occhi is research and development manager, and Micaëla B. Raschle is the marketing manager, all at Exalos AG in Zurich, Switzerland; e-mail: [email protected].