Superconductor Probe Results in Shock
ITHACA, N.Y., Aug. 4, 2006 -- High-temperature superconductors, which conduct electricity without
resistance at relatively high temperatures, have been known for 20
years, but how they work is still a mystery. By observing events at the
atomic scale, researchers have now found evidence that the mechanism in
high-temperature superconductors may be much more like that in
low-temperature superconductors than was previously thought.
"This
came as a huge shock," said Cornell physics professor J.C. Séamus
Davis, who with colleagues reports the findings in the August 3 issue
of the journal Nature.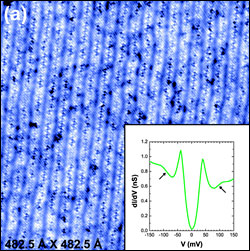
Scanning
tunneling microscope "topographic map" of a sample of cuprate
semiconductor shows the locations of atoms in the crystal lattice. The
inset shows how the current flow at a single point of the scan varies
with voltage, with "kinks" (arrows) that indicate the presence of
lattice vibrations and electron pairs. (Image: Davis Lab/Cornell
University)
The new research may shed light on how
superconductivity works in modified copper oxides known as cuprates,
which superconduct at the relatively "high" temperature of liquid
nitrogen. "The main expectation has been that electron pairing in
cuprates is due to magnetic interactions. The objective of our
experiment was to find the magnetic glue," Davis said.
Instead,
the researchers found that the distribution of paired electrons in a
common high-temperature superconductor was "disorderly," but that the
distribution of phonons -- vibrating atoms in the crystal lattice --
was disorderly in just the same way. The theory of low-temperature
superconductivity says that electrons interacting with phonons join
into pairs that are able to travel through the conductor without being
scattered by atoms. These results suggest that a similar mechanism may
be at least partly responsible for high-temperature superconductivity.
"We
have shown that you can't ignore the electron-phonon interaction,"
Davis said. "We can't prove that it's involved in the pairing, but we
have proven that you can't ignore it."
The superconducting
phenomenon was first discovered in metals cooled to the temperature of
liquid helium, about 4 K (4 ° above absolute zero, -270 °C or -452 °F).
Cuprates can become superconductors up to about 150 K (-123 °C or -253
°F). They are widely used in industry because they can be cooled with
liquid nitrogen (boiling point 77 K), which is less expensive than
liquid helium.
Drawing on a technique developed at Cornell a
decade ago to measure the vibrations of a single atom, Davis extended
the measurements across an entire sample, using an improved scanning
tunneling microscope (STM). The STM uses a probe so small that its tip
is a single atom; positioned a few nanometers above the surface of a
sample and moved in increments smaller than the diameter of an atom, it
can scan a surface while current flowing between the tip and the
surface is measured.
For the experiments reported in Nature,
the researchers examined bismuth strontium calcium copper oxide, a
cuprate that superconducts below 88 K. At each position in their scan
they conducted several measurements, including one to detect the
presence of paired electrons and one to show the presence of vibrations
in the crystal lattice. Each of these appears as a "kink" in current
flow as voltage is increased. "We simultaneously see lattice vibrations
with which clouds of electrons are associated," Davis said.
The
researchers found the same to be true with a variety of different
"dopings," in which atoms of other elements are inserted into the
crystal to create "holes" where electrons are missing. Since the holes
change the magnetic fields in the crystal, this suggests that magnetic
effects are not an explanation for the electron pairing, they said. On
the other hand, making the cuprate sample with a different isotope of
oxygen -- one with an atomic weight of 18 instead of 16 -- changed the
magnitude of the results, reinforcing the idea that the pairing relates
to vibrations of the atoms.
"A direct atomic scale influence
of (lattice vibration energy) on (electron pairing energy) is implied,"
the researchers conclude in their paper. Lead authors of the paper are
Cornell researcher Jinho Lee and Kazuhiro Fujita, visiting from Tokyo
University. Professors S. Uchida of Tokyo University and H. Eisaki of
AIST Labs, Tsukuba, Japan, provided the isotope substitution. Davis
also collaborated with other scientists in Japan and at the Los Alamos
National Laboratory.
The work was funded by Los Alamos, the Office of Naval Research and Cornell. For more information, visit: www.cornell.edu
/Buyers_Guide/US_Naval_Research_Laboratory/c10085