Immersion probes offer advantages for testing powders, solids, slurries and liquids.
BRIAN MARQUARDT AND GIORA PROSKUROWSKI, MARQMETRIX INC.
Since its inception in 1928, Raman spectroscopy has allowed scientists to see a chemical “fingerprint” of material. Using light, substances are identified by the way specific wavelengths interface with a sample and are scattered. Pioneered by Sir C.V. Raman 90 years ago using simple sunlight with a series of filters to read a sample, Raman spectroscopy has evolved to become an information-rich measurement technique.
The advent of the laser in the 1960s significantly boosted the effectiveness and sensitivity of Raman spectroscopy, however not without challenges still present. Raman is a weak effect and the challenge has been separating the inelastically scattered light from the intense Rayleigh-scattered laser light. To overcome this, modern instrumentation utilizes high-performance edge filters and notch filters for laser rejection. Additionally, because of the weak effect, Raman features can be masked by photoluminescence; however, scientists have found that utilizing multiple laser excitation systems to switch between excitation wavelengths optimizes Raman features when fluorescence may be present.

A powder measurement, as shown here, can be traditionally challenging for Raman measurement as the particle size can impact the measurement setup. With a spherical sapphire lens, there is no need to change accessories for different substrates, yet the user will achieve equal performance. Courtesy of Gloria Proskurowski, Marqmetrix.
Other challenges with Raman spectroscopy are due mainly to optical sampling. In some cases the sampling container or slide will interfere with the measurement, returning spectra for the container as opposed to the intended sample. When utilizing higher-power lasers to increase the Raman signal, the sample can be damaged. All types of samples have a laser power density threshold, and operating below that threshold will protect the sample integrity and the resulting measurements. While Raman measurements can be made with any excitation source, standard wavelengths for Raman are 532, 633, 785, 993 and 1064 nm, primarily determined by the common availability of stable lasers at those wavelengths.
Even with the aforementioned challenges, Raman remains a robust measurement technique for accurate identification and quantification. Unlike most spectroscopic methods, Raman can be a quantitative technique, as the amount of inelastically scattered light is directly related to the concentration of the analyte causing the scattering. Another powerful aspect of Raman spectroscopy is that water is a relatively low Raman scatterer, meaning that the signal from water has minimal effect on the analyte signal. With the right sample interface, the Raman technique is largely insensitive to sample type, so no sample handling or preparation is required. Lastly, Raman is nondestructive; the sample is not consumed during the measurement process.
Traditional Raman measurements require the user to gauge and adjust the focal distance, which can lead to variation in the measurement results. For example, a scientist would move the sample up and down, much like a microscope platform, until the signal was maximized. Even with proper training and equipment, this focusing sequence can be a subjective process requiring active decisions leading to unavoidable sampling reproducibility errors.
Another focusing technique involves using a visual imaging camera with the probe. With this technique there is a camera imaging the object or sample while the user adjusts the sample position into focus visually. Once focus has been achieved in the camera image, a Raman measurement is performed. The challenge with this method is that many times the focal distance of the camera is not the same as the focal point of the Raman laser. In effect, this technique still requires the operator to manually focus before each measurement.
Eliminating sampling error
Recent advancements in Raman instrumentation include probes that feature a spherical lens. With any data measurement process, reliability and repeatability are paramount and something scientists are always keen to improve. Reliability of Raman measurements is dependent upon the laser power density; adding curvature to the instrument lens focuses the laser into a tighter area after it is fired. Originally, when developing the spherical lens Raman probe, the goal was to concentrate the laser for tight focus. During development, it became apparent that sampling errors due to the variable focal distance could be eliminated by touching the lens directly to the sample. And because of the curvature, the “touch” takes place at the tangent of the sphere, exactly where the optical focus is located.

Today’s Raman instrumentation provides accuracy, versatility and flexibility for capturing qualitative and quantitative data and can be used in many different environments. Courtesy of Gloria Proskurowski, Marqmetrix.
The lens material also needed to be durable and resistant to scratching for the probe to work in “touch” mode. Therefore, sapphire spherical optics were employed because sapphire is one of the hardest optical materials, second only to diamond. The challenge was finding optically pure sapphire free of impurities that can have intense Raman signals that can interfere with sample measurement. UV-grade sapphire offers the lowest levels of chromium and titanium impurities on the market.
With the combination of a spherical lens and sapphire material, the measurement-to-measurement reproducibility has been demonstrated to less than 0.1 percent relative standard deviation when measuring titanium dioxide concentration in a latex paint application. These results were considerably less than the sampling error, ~2 percent, calculated when focal adjustments were performed manually.
The optical train, including the lens, fixes the focal length at the tangent of the sphere, regardless of the sample type or sample form. This leads to a Raman measurement device where the user simply has to touch the sample to take the measurement.

A recent advancement in Raman instrumentation is the MarqMetrix TouchRaman BallProbe, which features a spherical lens, shown above. The lens is made with UV-grade sapphire for its rugged interface and purity. Courtesy of Gloria Proskurowski, Marqmetrix.
Testing powders, slurries and liquids
Raman spectroscopy has had historical challenges with efficiency and accuracy across different sample types partially because samples had to be pure or in a single state to mitigate interference and produce repeatable measurements. Immersion probe technology was designed to address these issues, broadening the value of use across different sample mediums, such as powders, solids, slurries, liquids and gasses, specifically to mitigate error and improve reliability of the measurement.

Looking at liquids specifically, Raman BallProbe technology is valuable for analyzing heterogeneous solutions. As long as the mixture is touching the spherical lens, the user will obtain accurate and precise sample data. In the case of small volume samples, the spherical lens probe is effective at measuring the sample contents without interference from the container — for example, well plates and low-volume vials. Granular materials and powders can be especially challenging for traditional standoff Raman measurements as particle size can impact the measurement setup. The common approach is to require the user to fill a glass vial with the powder and measure through the glass.
With the spherical sapphire lens, there is no need to change accessories for different substrates, and the user will achieve equal performance. Additionally, the touch interface allows the laser to lead directly into the sample, mitigating scatter. The sphere generates the focus right at the tangent of the optic. So while a flat window probe would work well in immersion, the measurement result would vary quite dramatically when measuring a powder due to light scattering in different directions. Traditional flat-lensed Raman probes typically include several accessories that must be used to accommodate measurements of various sample types, such as a vial holder for powders, a fixed focal spacer for solids and a sealed optic for immersion.
With the advancements of the spherical sapphire lens in probes, measurement is simplified to “if a user can see and touch the sample, they can measure it,” without having to adjust the probe type or the focal distance. The probe separates the scientist from the measurement, improving sampling and measurement reproducibility.
No fouling of the device
The spherical interface of this technology minimizes fouling or sample buildup. Unlike flat optics where fouling begins at the edge of the optic and grows into the center, the spherical lens on the probe is self-cleaning at the tangent of the sphere — right where the measurement occurs. This technology has made Raman more accessible for process sampling and in situ measurements.
Spherical probes have been installed in process environments that are challenging with respect to flow and fouling, including wet granular materials and fluidized beds. In one case, the device ran continuously for 18 months without maintenance after replacing a traditional probe that fouled within two weeks of initial installation.
Taking it to the field
Not all measurements can take place in a laboratory or controlled setting and the benefits of Raman spectroscopy should not be limited to the lab. Challenges with suboptimal measurements — even when using optical spacers or adapters to control focal distance — have discouraged
the use of the technology. Raman was often shelved or not used in these applications because it was too time-consuming to ensure the best measurements. A recent user of a quality control application in cosmetics nearly abandoned using Raman because the technique did not meet the needs of the company when implemented outside the R&D laboratory. When the BallProbe was installed as the sample interface, the production line results began to match the expectations developed by the R&D team.
Another challenge in the field can be extreme conditions, especially when considering the petrochemical and oil and gas industries. Developing a high-pressure probe that can withstand 20,000 psi and 500 degrees Celsius was a logical evolution once it was realized that the sapphire lens could withstand and operate effectively in extreme conditions. Probes are available for use in high-pressure (up to 25,000 psi) and temperature applications.
Greater flexibility, broader applications
Raman spectroscopy is a superior measurement technology because of the flexible sampling interface, high sampling rate and high spectral specificity. Today’s Raman instrumentation provides accuracy, versatility and flexibility for capturing qualitative and quantitative data. It can be used in many different environments as an analytical tool for the study of solids, powders, slurries, liquids and gases. A Raman instrument interfaced with a fiber-coupled probe has the versatility and flexibility for measurements to be made in situ for the monitoring of processes.
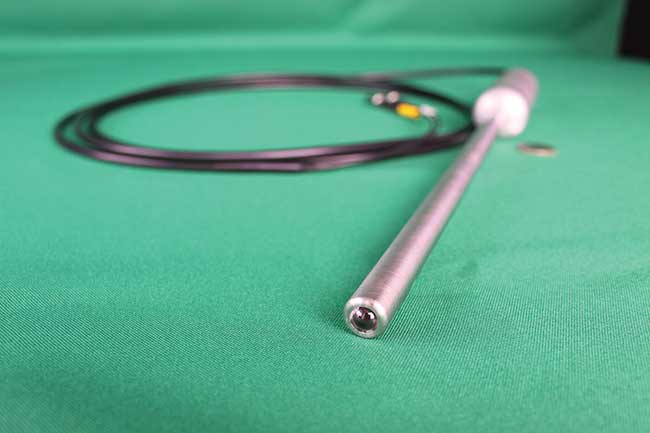
A Raman instrument interfaced with a fiber-coupled probe, as shown here, given it the versatility and flexibility for measurements to be made in situ for the monitoring of processes. Unlike flat optics, where fouling is a challenge, the spherical interface minimizes sample buildup.
An instrument with a sapphire sphere as the measurement interface is a recent technological advancement that further improves the usability and accessibility for Raman measurement. New applications for the technology because of the rugged interface and reliability include measuring hydrothermal vents at the bottom of the ocean to high-temperature, high-pressure environments in the oil and gas industry. When working with high-value materials and small sample sizes, such as in pharmaceuticals, the anti-fouling properties and ability to measure in-situ mitigate waste of raw materials, providing a more valuable measurement option. New applications are being developed and technologies continue to advance in the world of Raman.
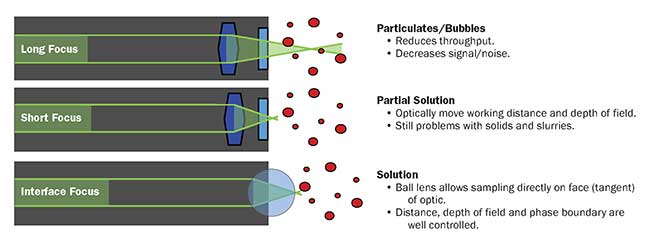
Meet the authors
Brian J. Marquardt, Ph.D., is the co-founder of MarqMetrix, an optical sensor and instrumentation company focused on industrial process measurement and control. He is also the director of the Center for Process Analysis and Control and senior principal engineer at the Applied Physics Laboratory at the University of Washington. Research interests include the development and application of spectroscopic instrumentation, primarily Raman and LIBS, for continuous real-time chemical, biological and environmental analysis.
Giora Proskurowski, Ph.D., is the director of MarqMetrix Raman Applications and Probes, which includes applications development and management of the BallProbe product line. Before joining MarqMetrix in 2014, he spent a decade working on oceanographic instrument development, production and deployment.
His research focused on the biogeochemistry of deep-sea hydrothermal vent volatiles and the distribution and chemistry of plastic marine debris.