When Sir Isaac Newton discovered that the white light from the sun could be dispersed into a continuous series of colors — a phenomenon he described as a “spectrum” — he essentially started the engine for the science of spectroscopy.
Today, resulting technology is helping examine the far reaches of the universe and look deeper inside the human body, and is making its way into new devices. Some of the latest advancements are in biomedicine, according to Robert Alfano, distinguished professor at The City College of New York and director of its Institute for Ultrafast Spectroscopy and Lasers (IUSL). He and Lingyan Shi, a research associate at the institute, told Photonics Spectra about the breakthroughs they’re seeing — and helping to drive — in spectroscopy today.
Resonance Raman scattering, as well as second harmonic generation and two-photon excitation fluorescence microscopy are allowing for greater inspection of the various stages of cancer, and deeper, as well as noninvasive, imaging
of the brain and potentially other biological tissue. Spectroscopy advances are leading experts to envision a day when the technique will complement the more traditional — and more expensive — technologies used for imaging, such as PET and MRI.
Photonics Spectra: We have been reading about your team’s work with longer wavelengths in the near-infrared (NIR), and its promise for future noninvasive, deep imaging of biological tissue. What do findings such as this mean for biological imaging and spectroscopy research overall?
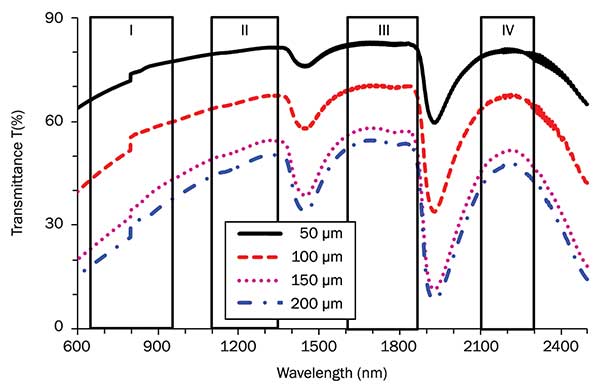
Transmittance T (%) in optical tissue windows I, II, III and IV. Brain tissue es were 50, 100, 150 and 200 µm, respectively. Photo courtesy of Lingyan Shi and Robert Alfano.
Dr. Shi led the team to examine absorption in rat brain tissue in the NIR range (700 to 2500 nm). She found that the optimal NIR window for penetrating deep into the brain tissue is between 1600 to 1870 nm, which is called optical window 3, also named the “golden window.” This wavelength region is ideal for nonlinear optical imaging processes such as two- or three-photon excitation fluorescence [2PEF, 3PEF] and second harmonic generation [SHG] to image deeper into brain tissue. As
shown by [her] publication in the [Journal of] Biophotonics, [doi: 10.1002/jbio.201500192, January 2016], there are four optical windows in NIR, and to obtain deep imaging of biological tissues, the excitation and emission wavelengths should be in one of these optical windows. An optimal case is excitation wavelength in window 3 (golden window) and emission in window 1 (700 to 900 nm). Reducing scattering in NIR windows reduces blurring of images for deeper penetration.
The finding of the golden window is a major leap for deep imaging into brain tissue without removing the skull, therefore promising for future noninvasive deep imaging into other biological tissues.
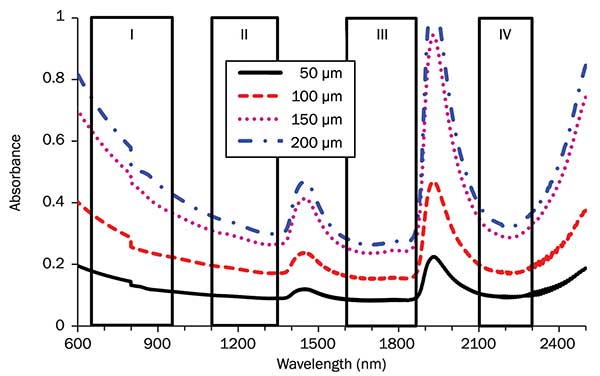
Absorbance in optical tissue windows I, II, III and IV. Brain tissue thicknesses were 50, 100, 150 and 200 µm, respectively. Photo courtesy of Lingyan Shi and Robert Alfano.
PS: In what specific areas of spectroscopy are you seeing the most advancement? What is the most exciting work you’re seeing?
The most advancements in spectroscopy, I think, are in two areas: [One is] Resonance Raman scattering (RRS), using the magic laser wavelength at 532 nm to generate vibrations that are associated to flavins in tissues. The strength of [the RRS] signal is stronger than the commonly used 785 nm laser, because 532 nm is in the spectral wing of [the] flavin absorption and in fluorescence spectrum of the tissue, the Raman cross-section is enhanced, and the background noise from wing in Raman scattering is nearly gone. One can obtain Raman spectra within almost 0.1 second.
[The second area of advancement is] using second-harmonic generation (SHG) and two-photon excitation fluorescence (2PEF) microscopy with sub-micron resolution for deep imaging in brain and other tissues.
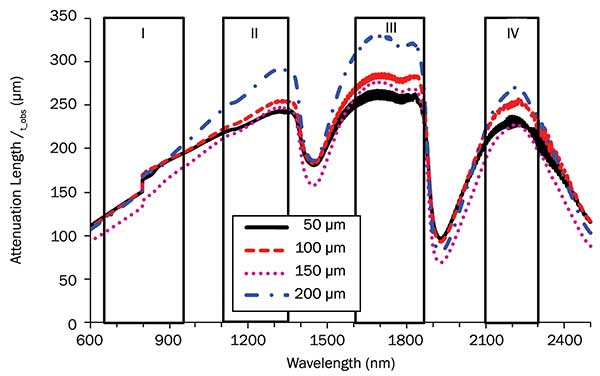
Spectra of the total attenuation length in optical tissue windows I, II, III and IV. Brain tissue thicknesses were 50, 100, 150 and 200 µm, respectively. Photo courtesy of Lingyan Shi and Robert Alfano.
The most exciting work[s] I am seeing are the applications of [RRS] with a 532-nm laser in investigating vulnerable plaque in arteries and in examining stages of brain, skin and breast cancers, and the application of 2PEF microscopy, combined with ultrasound sonication and microbubble, for studying drug delivery across the blood-brain barrier.
PS: What do you anticipate for the future of spectroscopy — in research, as well as in development of novel devices/systems? Is there a particular unmet need?
Over 10 years ago a compact photonic explorer (CPE) (Biomedical Microdevices, [doi: 10.1007/s10544-005-1588-x], 2005) was introduced to image in the body using optical spectroscopy (excitation, fluorescence and Raman), like that played in
the movie “Fantastic Voyage,” where a small ship enters into the human body.
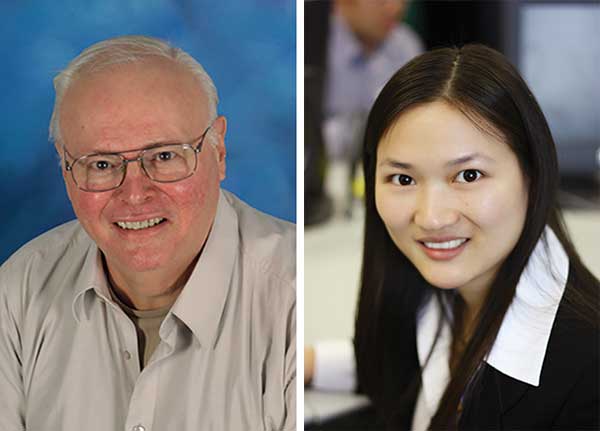
Robert Alfano and Lingyan Shi.
A take-off was the Givens Pillcam, which was the first step to take visual photos down the gastrointestinal (GI) [tract] using a CCD camera and send them back wirelessly.
With the advent of smaller CMOS imaging detectors, blue LEDs, advanced batteries, small microprocessor circuits, cellular phone technology and advanced computer chips, a quantum leap of developing better CPE on millimeter-scale,
optical spectroscopic-based robots to travel in GI and urinary tracts is ahead of us to diagnose cancer and transfer the information to computers wirelessly.
One can envision smart spectroscopy, photonic-based endoscopes and devices for a new medical armamentarium to complement the visual inspection from more expensive PET and MRI images.