Researchers are lowering costs and improving efficiency.
Michael A. Greenwood, News Editor
Throughout its long history, Japan has been known as the land of the rising sun. A modern adaptation of that moniker might be the land of the multiplying solar panels.

The roof of Terminal 2 at Munich International Airport in Germany was outfitted with solar arrays in 2002. The arrays are designed to tilt toward the sun to maximize energy production. Courtesy of BP Solar.
The island nation has embraced the sun’s considerable power and continues to lead the world (along with Germany) in the use of the clean, ever-renewable and, some would argue, perfect energy source.
But although the power of the sun is almost unlimited — it provides nearly as much energy in one hour at the Earth’s surface as the total amount of energy consumed in a year — solar power today remains relatively untapped, a niche technology even in the most photon-drenched corners of the world (Figure 1).
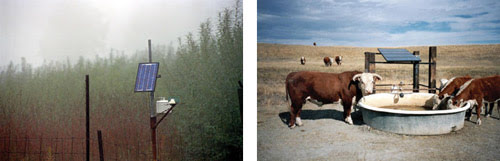
Figure 1. A weather station uses photovoltaic power to transmit information such as temperature and wind speed in Tully, N.Y. (left). In Oelrichs, S.D., water is pumped into a livestock tank with photovoltaic power (right). Courtesy of US Department of Energy/National Renewable Energy Laboratory.
The US, known for a ravenous energy appetite, produced about 479 MW of solar power in 2005. Japan, by comparison, produced 1422 MW (Figure 2). The US output represents less than 1/10 of 1 percent of its overall energy consumption. In other words, solar power generated in the US is capable of providing for the energy needs of about 100,000 houses, according to the National Renewable Energy Laboratory in Golden, Colo. Most all other countries are far behind even that humble output (sun-baked Spain, for instance, produced only 57 MW).
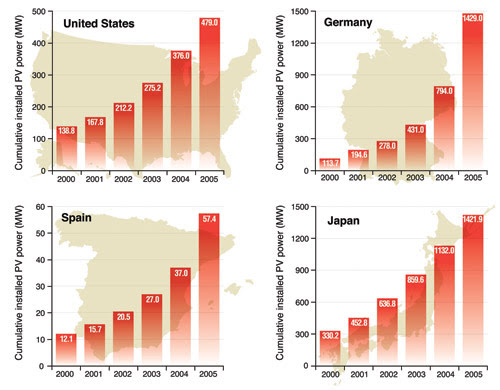
Figure 2. The amount of installed photovoltaic (PV) power has grown dramatically in some countries in the first part of the decade. Courtesy of National Renewable Energy Laboratory.
But harnessing photons and efficiently and economically converting them into energy remains a technological challenge. Approximately 90 percent of the photovoltaic systems used today, and most likely into the foreseeable future, are and will continue to be made of silicon, a versatile substance that has many shortcomings. Several other nascent solar technologies — thin films, plastics, multijunction concentrators and even quantum dots — have their own mixed résumé of strengths and weaknesses. Traditional carbon-based sources of power in many cases remain more convenient and less expensive. As a result, relatively few people have embraced solar power.
However, ominous warnings about global climate change, compounded by political discord in some oil-producing regions of the world and the spiking cost of conventional fuels, have prompted many people to look with renewed interest at the sun. Indeed, this slowly maturing technology is poised to blossom in coming years into a significant power source.
Solar shortcomings
If solar power is so great, why isn’t its use already more widespread? The basic answer is quite simple. This very modern technology continues to be beset by some very traditional problems.
“It’s always a challenge of efficiency and cost,” said Martha I. Symko-Davies, a research senior supervisor with the National Renewable Energy Laboratory.
Most photovoltaic technologies operate under the same general principle. Each cell consists of two semiconducting materials, creating a positive and a negative layer. When the cell is struck by a photon with the appropriate energy, electrons are knocked free and their movement from one layer to the other generates electricity.
Existing technologies exploit this phenomenon with varying degrees of success. Silicon photovoltaic cells have been improving gradually and, in ideal conditions, now can achieve more than 20 percent efficiency, with roughly one-fifth of the sunlight hitting the photovoltaic cell converted into electricity. Thin-film technologies also have been inching upward in performance and today offer cell efficiencies in the mid- to high teens.
Newer approaches, such as plastic cells, still lag well behind, having achieved efficiencies in the range of about 5 percent. A fourth approach, multijunction concentrators, would seem to be the obvious choice. This technology recently achieved a once-unimaginable efficiency of more than 40 percent. The problem with this type of cell is that it is too expensive for widespread use.
Considering that photovoltaic systems are put out of action every time night falls, achieving the highest possible efficiency is critical for solar power’s viability.
Cost remains the other bugaboo that has slowed the technology’s spread. Many systems continue to be expensive to build, install and maintain. Although prices have trended gradually downward in recent years, solar power remains a pricey proposition for many.
In 2005, for instance, photovoltaic systems for residential use ranged from 23 to 32 cents per kilowatt-hour. For commercial applications, the cost was between 16 and 22 cents. By 2015, the Solar America Initiative (a program created in 2006 by the US Department of Energy to advance the use of solar power) wants to cut these costs by more than half.
Its target is for residential solar power to cost between 8 and 10 cents per kilowatt-hour and 6 and 8 cents for commercial uses. Electricity, by comparison, currently costs from 6 to 17 cents per kilowatt-hour for residential customers and approximately 5 to 15 cents per kilowatt-hour for commercial customers.
Reducing the cost of photovoltaic systems will be accomplished in part by science, through advances in efficiency and improved materials. Education and political support are the other parts of the equation.
“It’s an incredible resource,” said Thomas Surek, photovoltaic program manager for the National Renewable Energy Laboratory. “But we have to learn to capture it cheaper.”
Researchers at universities and energy companies are developing a variety of novel techniques to achieve just that goal.
Extracting efficiency
Researchers at Georgia Tech Research Institute in Atlanta have developed a 3-D thin-film system designed to trap incoming photons until they are absorbed and converted into electricity. It has been likened to a “nano-Manhattan.”
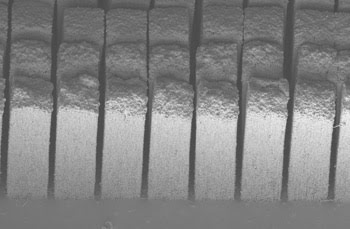
Figure 3. An array of carbon nanotubes is shown as it appears under a scanning-electron microscope. Each tower is approximately 100 μm tall, and the towers are about 10 μm apart. Courtesy of William J. Ready.
The system features row upon row of carbon nanotube arrays coated with the semiconducting materials cadmium telluride and cadmium sulfide. When examined under a scanning electron microscope, the setup indeed resembles the skyscrapers that punctuate Manhattan’s skyline (Figure 3). The intention is for photons to become ensnared in the tiny chasms between the nanotubes, ricocheting wildly through the streets of the Lilliputian city until they are eventually absorbed (Figure 4). This differs from the traditional planar approach, where photons have one chance to interact with the 1-D solar cells. Some are absorbed, but most are reflected by the flat surface — and thus wasted — in the process.

Figure 4. An array of carbon nanotubes offers multiple chances to absorb an incoming photon. CNT = carbon nanotube; TCO = transparent conductive oxide. Courtesy of William J. Ready.
William J. Ready, the project’s senior research engineer, said his ultimate goal is to tap the power of every photon. His system was tested against a standard silicon planar cell, and the results were reported in the March issue of the Journal of the Minerals, Metals & Materials Society. In terms of efficiency, the planar system produced 0.7 mA/cm2. The 3-D nanotube approach, by comparison, achieved 44.4 mA/cm2, a sixty-threefold improvement in production.
Ready’s nanotubes, created by molecular beam epitaxy, measure 100 × 40 × 40 μm and are set about 10 μm apart. This approach to manufacturing solar cells could mean smaller arrays, which would mean less weight, less space taken up and, ultimately, less expense. Ready said that additional work is needed to determine the ideal dimensions of the nanotubes. It will be at least five years before this technology will be available for general use.
Plastic payoff
In nearby North Carolina, David L. Carroll also is seeking to improve solar efficiency — with plastic.
His team of researchers at Wake Forest University in Winston-Salem reported in Applied Physics Letters, published April 16, an efficiency of 6 percent, a record for this relative newcomer to the field of solar materials. Just two years ago, efficiency for plastic cells was about 3 percent. Carroll, an associate professor of physics, believes that 10 percent can be attained by 2008.
Plastic (also known as organic or polymer) cells have a number of advantages — and disadvantages. One disadvantage is glaring: They are nowhere near as efficient today as their silicon and thin-film cousins. But plastic cells offer some tantalizing benefits: They are cheap (potentially pennies per kilowatt, a level at which solar power would be an option for many more people), they are much lighter than bulky silicon modules, and they are highly flexible — enabling aesthetic integration onto houses and even cars.
“Our cells can look like traditional building materials, whereas the silicon panels cannot,” Carroll said.
If efficiency can be further enhanced, plastic solar cells have the potential to be commercially viable beyond the very few specialty markets they currently serve.
Carroll’s research team used a thermal annealing technique to create a delicate network within a light-absorbing plastic (Figure 5). The effect is like veins of a leaf. To achieve still better results, Carroll said that the plastic’s band of absorption will have to be broadened. Right now, only a small portion of the incoming light is getting through. The researchers are seeking to integrate frequency conversion into their photovoltaic system, in effect changing the color of incoming sunlight so that it can be absorbed.
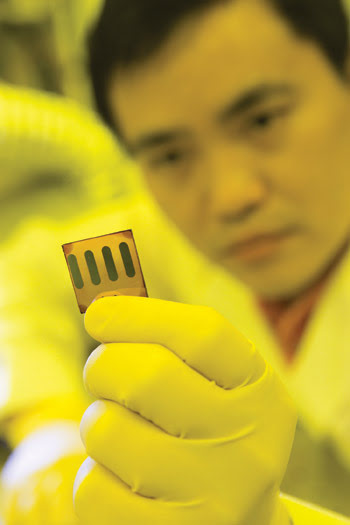
Figure 5. Jiwen Liu, a researcher in the Wake Forest University Center for Nanotechnology and Molecular Materials, tests a new solar cell in the center’s laboratory in Winston-Salem, N.C. Courtesy of Wake Forest University.
Yang Yang, a professor and researcher at the University of California, Los Angeles, also is working to improve plastic’s performance. He has achieved 4.4 percent conversion efficiency and believes that by using different materials with better light-absorption abilities, that figure will be doubled. He is seeking to create a polymer with a smaller bandgap that picks up more photons. There is currently no such material that simultaneously satisfies three crucial parameters: bandgap, the position of energy bands and carriers’ mobility, he said.
Yang ultimately wants to achieve efficiencies for plastic cells in the range of 15 to 20 percent with a life span of at least 10 years.
Dots and electrons
Quantum dots — semiconductor nanocrystals generally less than 10 nm in diameter — already are used as a light source in a number of applications. Some researchers believe that quantum dots also have a bright future in harvesting light (Figure 6).

Figure 6. Semiconductor quantum dots (or nanocrystals) are displayed in a hexagonal close-packing arrangement. Arrays of quantum dots are being studied for their photovoltaic capabilities. Courtesy of Randy J. Ellingson.
In most photovoltaic systems, one absorbed photon creates one electron-hole pair (also called an exciton). But because of a quantum dot’s minuscule size, a single absorbed photon can generate multiple electron-hole pairs. This phenomenon is known as multiple exciton generation and has been observed in quantum dots made of a variety of materials, including silicon. A photon with double the bandgap energy of a quantum dot can produce two electron-hole pairs, and a photon with three times the bandgap energy can produce three. This unique response could significantly improve the efficiency of solar cells.
Randy J. Ellingson, a senior scientist and member of a team that is working on advanced concepts for photovoltaic cells at the National Renewable Energy Laboratory, said that, if the process of multiple exciton generation can be fully controlled and optimized, quantum dots could achieve efficiencies as high as 44 percent.
“Such a technology would greatly improve the prospects for solar energy to become economically competitive,” he said.
But technological bugs remain to be ironed out. One of the most basic problems is integrating quantum dots into solar cells. The large number of interfaces between the quantum dots and the collecting electrodes interferes with the smooth transfer of the charge. As a result, many electrons return to their holes before their power can be harvested. One important aspect of the national laboratory’s research is focused on configuring the quantum dots in various solar cell designs to dissociate the excitons and efficiently extract the extra charge carriers. Ellingson estimates that it will take from three to seven years before quantum dot solar cells become commercially important.
Solar breakthrough
Spectrolab Inc. of Sylmar, Calif., is in an enviable position. It has been breaking its own photovoltaic records. Another benchmark was achieved in late 2006 when the company, a subsidiary of Boeing Co., reached an efficiency of 40.7 percent with a multijunction solar cell. Tests measuring power output duplicated the cell’s performance as if the cell were installed in a solar concentrator. It is believed to be the highest efficiency achieved for any type of photovoltaic system. It is also one of the more complicated approaches, though capable of generating renewable energy at costs that are projected to be competitive with the prices of energy derived from fossil fuels.
Unlike most other approaches to solar cells, which usually have a single silicon junction, the multijunction technique features three photovoltaic layers, one stacked upon the other, each with different bandgap energy. The result is that more of the available light is captured and converted more efficiently.
The record-breaking cell consisted of gallium indium phosphide (good for the short-wavelength portions of the spectrum), gallium indium arsenide (the middle part of the spectrum) and germanium (which captures energy from the IR). The breakthrough in efficiency was a result of the construction of the cells. They were produced in a metamorphic fashion — also known as lattice mismatch — which allowed energy bandgaps to be manipulated so that each subcell was more efficiently matched to the spectrum. The cells were further enhanced by solar concentrators that focus the sunlight — much like a magnifying glass — with the intensity of 240 suns to increase power output. The company’s concentrators are made from less expensive mirrors and lenses, rather than of more expensive semiconducting materials to reduce costs.
Multijunction solar cells were developed primarily for powering spacecraft. But researchers believe that the concentrators make the device more affordable, and they are starting to appear in terrestrial markets. In Hermannsburg, Australia, for instance, approximately 1500 of the company’s multijunction concentrator cells are being tested. The receiver is delivering power in excess of 30 kW, almost 50 percent more than could be achieved with silicon concentrator cells.
The future of solar
And progress is not likely to stop at the existing benchmark of 40.7 percent. The company is conducting experiments with four junctions, metamorphic materials and other design approaches that could achieve efficiencies of 45 percent. It is now mass-producing and shipping concentrator solar cells with efficiencies of about 36 percent and hopes to be shipping solar cells with efficiencies of nearly 42 percent by 2010.
Government is proving to be one of solar power’s biggest fans. Its role in developing the technology and encouraging its use can readily be seen in countries such as Japan and Germany. Other nations are starting to follow their examples.
Although spending hasn’t exactly been lavish, the US government, along with various state governments such as California, New York and New Jersey, is starting to devote more resources to solar energy in the form of financial incentives and public policy.
The Million Solar Roofs initiative was launched during former President Bill Clinton’s administration to sow interest in solar power. And although the agency never outfitted a million roofs with solar, it succeeded in forming hundreds of partnerships with states, utilities, businesses and builders that helped jump-start the budding technology.
That effort is now being orchestrated by the Solar America Initiative, which has established ambitious goals for solar power usage in the US. In a best-case scenario, the group forecasts that the country will have 10 GW of solar power installed by 2015, an exponential increase over current levels. By 2030, the level could be as high as 100 GW.
“I think it is inevitable that solar will play a big role,” Thomas Surek of the National Renewable Energy Laboratory said. “Solar must be part of the solution.”
Roger Little, president and CEO of Spire Corp., a Bedford, Mass.-based manufacturer of turnkey photovoltaic factories that are currently operating in 52 countries, is confident that solar energy will become increasingly important.
He said that the current shortage in the silicon supply has done little to slow business and predicts that the shortage will evaporate by next year and that demand for photovoltaic systems will expand at a breakneck pace thereafter.
“We can barely keep up,” Little said. “It’s exploding worldwide. The US has become the next big market. It’s going to grow like crazy.”
Nature's Solar Cells Set the Standard
When it comes to devising solar cells, mankind’s best efforts are still dwarfed by the designs of Mother Nature. Plants have long been known for their ability to harvest the sun’s energy through photosynthesis, squeezing energy out of essentially every photon absorbed.
A complete understanding of nature’s near-perfect method has remained elusive. But researchers at Arizona State University in Tempe moved a step closer with a recent study of the mechanisms that drive photosynthesis, findings that could have implications for the design of man-made solar cells. The results are reported in the May 4 issue of Science.
The researchers, led by Neal W. Woodbury, focused their study on the business end of solar energy capture — the reaction center — in a photosynthetic bacterium. To better understand how it works, they created mutations in the bacterium that would change how the electron transfer between molecules in the reaction center occurred.
Using an ultrafast mode-locked Spectra-Physics Ti:sapphire laser for femtosecond transient absorption spectroscopy, the scientists measured the extremely fast protein movement in the reaction center and matched this movement to how the electrons were transferred in the center. They discovered that, although the mutations they induced made it harder for photosynthesis to happen, proteins within the reaction center moved to compensate and thus were able to still guide electrons correctly.
Better understanding the design of nature’s solar cells could be used to boost the performance of organic photovoltaic cells. Woodbury said that perhaps polymeric solvents could be incorporated into these cells to allow them to perform under changing conditions.
Nanowires and Nanocrystals Used to Construct Solar Cell
At the University of Minnesota in Minneapolis, researchers have created photovoltaic solar cells with semiconductor nanocrystals (or quantum dots) arranged like holiday ornaments on nanowires.
The researchers, led by David J. Norris and Eray S. Aydil, believe that quantum dots potentially have distinct advantages over photosensitive dyes in the construction of solar cells. They have a superior ability to match the solar spectrum because their absorption spectrum can be tuned with particle size. Quantum dots also have demonstrated an ability to generate multiple electron-hole pairs per individual photon, which could result in improved efficiency for photovoltaic cells. The researchers’ goal is to develop a high-efficiency, low-cost solar cell.
The photovoltaic cell was created with cadmium selenide quantum dots that were attached to the surface of single crystal zinc oxide nanowires. When illuminated with visible light, the quantum dots (~3 nm in diameter and absorbing light at 550 nm) injected electrons across their interface with the nanowires. These nanowires, ranging in length from 2 to 12 μm, provided a direct electrical pathway to the cell’s photoanode, which is believed to have improved electron transport. The results were published online in Nano Letters on May 16.
The nanowires were grown directly onto a substrate and immersed into a solution of quantum dots in methanol. To improve the coverage of quantum dots on the wires, the investigators treated the substrate with oxygen plasma before immersion.
The nanowire-nanocrystal device achieved short-circuit currents ranging from 1 to 2 mA/cm2 and open-circuit voltages of 0.5 to 0.6 V when it was illuminated with 100 mW/cm2. The scientists obtained internal quantum efficiencies as high as 60 percent, close to what similar nanowire dye-sensitized cells achieve. In terms of overall energy conversion efficiencies, however, the quantum dots achieved less than 1 percent, compared with a record 11 percent for dye-sensitized solar cells.
Further improvements in the efficiency and stability of the researchers’ prototypical structure will require the development of an optimal hole transport medium for the architecture. They are working on alternative architectures for their system and considering different materials for the nanowires and quantum dots. An optimized device would contain quantum dots of various sizes to absorb as much of the solar spectrum as possible.