MUNICH, Jan. 12, 2023 — A method of simulation of the additive manufacturing process could enable better yields and higher-quality results. Researchers from Fraunhofer member institutions combined different methods to develop a simulation of the 3D-printing process itself, at the microstructure level, in order to identify direct correlations between the workpiece properties and the selected process parameters.
For example, the additive manufacture of tools using a laser powder bed fusion (LBPF) process offers advantages; it is economical and precise, and it allows for customized solutions. However, it can be difficult to determine optimal process parameters involved with the method. These parameters include scan speed, as well as the power of the laser. Improving these parameters would in turn improve the quality of yields.
The graphic shows the simulation of the formation of a columnar microstructure in the laser melt pool. Collaborating researchers from Fraunhofer member institutions have demonstrated a simulation approach for the additive manufacturing process that targets the optimization of process parameters to improve yields and their quality. Courtesy of Fraunhofer IWM.
Claas Bierwisch, team leader at the Fraunhofer Institute for Mechanics of Materials (Fraunhofer IWM), said, “Because the laser powder bed fusion process is becoming increasingly complex due to new materials and requirements, we have decided to simulate the entire process chain. This enables us not only to minimize trial and error cycles, but also to quickly and effectively evaluate variations in the overall process and eliminate undesirable effects during manufacturing.”
It is important that components manufactured via the LBPF process have a density of 100% and no pores, and that each newly applied layer binds firmly to the layer below. This is achieved by adjusting process parameters such as scan speed and power of the laser. The microstructure of metallic grains is particularly important for the mechanical properties of the workpiece. These have certain orientations, sizes, and shapes and have a considerable impact on the mechanical properties, such as the material’s elastic modulus or the yield stress.
The researchers addressed the key question of how to control the manufacturing process in such a way that the resulting microstructure is suited to the component’s future conditions of use. Further, as components and workpieces are often made out of different metallic alloys and each alloy material has different properties and forms different microstructures, finding the optimal process parameters and materials and matching them to each other has to this point been an experimental and therefore time-consuming endeavor.

Using the discrete element method of simulation, the researchers first looked at how the individual powder particles are spread in the building chamber with the aid of a special tool, namely the doctor blade. They then looked at how the powder particles melt using the smoothed particle hydrodynamics method. Both the laser interaction and heat conduction are calculated, as well as the surface tension that causes the melt to flow. The calculation also accounts for gravity and the recoil pressure that occurs when the material vaporizes.
To predict mechanical material properties, the simulation must also describe the material’s microstructure.
Simulation of the LPBF process with lack of fusion defects and residual porosity. Courtesy of Fraunhofer IWM.
“To analyze this microstructure, we have incorporated another simulation method, known as cellular automation,” Bierwisch said. This additional method describes how the metallic grains grow as a function of the temperature gradient, according to Bierwisch.
Where the laser meets the powder, temperatures can reach up to 3000 ºC, though only a few millimeters away from this site, the material is cool. As the laser moves over the powder bed at a speed of up to several meters per second, the material heats up extremely quickly, but it also cools down again within milliseconds. All of this has an impact on how the microstructure is formed. The final step is the finite element simulation: The research team uses this to perform tensile tests in different directions on a representative volume element of the material to find out how the material reacts to these loads.
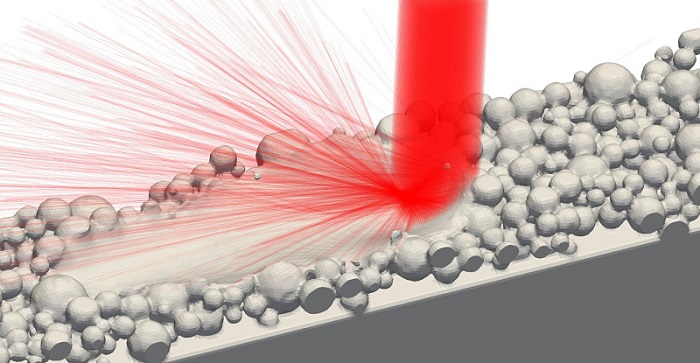
Raytracing simulation of the LPBF process. Courtesy of Fraunhofer IWM.
“In the experiment, we can only study the final result, whereas in the simulation, we can watch what happens in real time,” Bierwisch said. “In other words, we create a process-structure-property relationship: For example, if we increase the laser power, the microstructure changes. This, in turn, significantly affects the yield stress of the material. The quality of this is completely different to what is possible in an experiment. You can detect interrelationships in an almost investigative way.”