Marking and engraving industrial laser applications require specialized optics called scanning laser systems to achieve the required precision.
Michael R. McKinney, II-VI Inc., Michael R. McKinney is the graphic communications coordinator for II-VI Inc. in Saxonburg, Pa.
Think of industrial lasers, and you probably envision showers of sparks on robotic assembly lines as automobiles are welded, or on job-shop floors as sheets of steel are cut through like butter. No doubt, welding and cutting are among the world's top industrial laser applications.
Marking and engraving, the next-largest applications, operate on a much smaller and less dramatic scale. Driven to a great extent by government and industry regulations regarding product traceability for warranty and recall actions, they maintain an annual growth rate of more than 20 percent. Current systems can etch materials such as those used in integrated circuits at the rate of 1000 monochrome characters per second. Color marking and the ability to etch machine-readable bar codes on noncontrasting materials are under development.
To mark and engrave with such precision, a specialized series of optics guide the lasers. Collectively known as scanning laser systems, they comprise beam expanders, mirrors and scan lenses or lens arrays (Figure 1). Additionally, specially coated "band selective" resonator optics may be used to better process certain materials, such as plastics, using a CO2 laser.
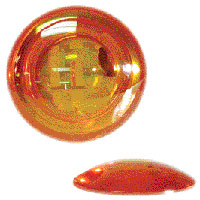
Figure 1. Together, beam expanders, galvanometer mirrors and lenses guide a laser beam to a work surface in marking and engraving applications.
There are several types of scanning laser systems, and each has its advantages and limitations. A preobjective scanning system is so-called because the mirrors that deflect the laser beam precede the scan lens or objective lens (Figure 2). These systems are excellent for marking and engraving metal, wood and plastic, where high-quality text or bar coding is required.

Figure 2. Preobjective scanning systems feature galvanometer mirrors before the lens. These systems are precise, but they scan over only a limited area on the workpiece.
Another type is the postobjective system, in which the mirrors are positioned after the focusing lens -- which may comprise two or three elements in a reverse-telephoto configuration -- to expand and refocus the beam. One of the lenses in the expanding section may be translated along the optical axis to adjust the focus position -- i.e., to flatten the focal plane -- as the mirrors scan the beam across the field. These systems have much larger field sizes --the area over which a system scans -- than preobjective ones. They also have larger focal lengths, which results in a larger focused spot size. Applications such as thin-material cutting and engraving over large-field areas, in which the linewidth may not be critical, are ideal for post-objective scanning.
A third type is the flatbed scanning system, which takes advantage of flying optics technology to move the beam in one or two axes. For those systems in which the optics move in one axis, the material usually is moved along a perpendicular axis so that two-dimensional processing is possible. These low-power marking and engraving systems have adopted their technology from the high-power CO2 flatbed metal-cutting and -welding systems. Like their high-power counterparts, they are ideal for extremely large field marking and engraving of materials such as wooden plaques and signs.
Each system may employ similar optics, or even the same optics in different configurations. The remainder of this article addresses those used in preobjective scanning systems: beam expanders, galvanometer mirrors, scan lenses and band-selective resonator optics.
Beam expanders
The focused spot size -- or final diameter -- of a laser beam on the workpiece is inversely proportional to the diameter of the beam that enters the scan lens. Because a smaller focused spot size provides greater precision, the larger the diameter of a laser beam entering the scan lens, the better. Beam expanders provide this precision by increasing the diameter before the lens.
They also produce a more tightly collimated laser beam. The divergence of the output from a beam expander is inversely proportional to the expansion ratio. If the diameter of an input beam increases by 300 percent, for example, the output beam will have one-third the divergence.
Expanding the beam requires larger optics and larger beam delivery tubes downstream, and larger optics cost more. Moreover, in the case of galvanometers, also called galvos, larger mirrors also limit the speed of the mirror rotation, which leads to slower materials processing speeds. Factors in determining the degree of beam expansion, therefore, include both the beam diameter and the galvo mirror aperture.
Most of the lasers used in marking and engraving systems have small beams (on the order of 2 to 4 mm in diameter) at their output. Most high-speed galvo mirrors have clear apertures in the 1- to 15-mm range. Consequently, beam expanders of 2X to 5X are most common.
Galvo mirrors
After a laser beam passes from the resonator and through the beam expander, it encounters two galvanometer mirrors. Galvanometers are spring-loaded, movable coils wrapped around a permanent magnet. When a current flows through the coil, the resulting magnetic field interacts with the permanent magnet and deflects the coil. Optical scanning systems that use these galvos provide the most precise scanning, but their field size is limited.
Affixed to stepper motors, these oscillating mirrors guide the laser beam into the scan lens along precise, two-dimensional X-Y coordinates. Lightweight substrates of mirror-grade silicon and fused silica are the best materials for repositioning these mirrors in fractions of a millisecond.
Galvo mirrors can be coated with a variety of metal/dielectric coatings to offer high reflectivity at various polarizations and wavelengths and increased laser power throughput. This also reduces absorptivity and, in high-power CO2 systems, thermal lensing. Because some marking and engraving systems use coaxial HeNe lasers or visible laser diodes to aid with the setup and alignment of the optical system, it is important to specify mirrors with good reflection properties at both the primary wavelength and at that of the alignment laser.
Scan lenses
The scan lenses focus the laser beam onto the workpiece. Unlike traditional focusing lenses that work primarily on-axis, scan lenses produce off-axis, or "flat-field," focusing. Regardless of the beam input/output angle and the distance from the lens to the workpiece (which increases as the field angle increases), the scan lens must maintain beam focus at the workpiece (Figure 3).
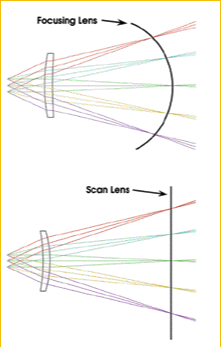
Figure 3. Focusing lenses work on-axis and can focus on curved surfaces. Scan lenses produce off-axis, or "flat-field," focusing.
For applications such as marking and engraving, a single scan lens, designed to provide a flat field, yields satisfactory results. However, other applications, such as electronics microvia drilling, require greater precision. To minimize spot distortion and drill angle, "telecentric" multielement lenses can accept a laser beam from varying input angles and focus it perpendicularly onto the work surface, regardless of the beam's position in the scan field (Figure 4).
As with the coatings for galvo mirrors, specifying the best antireflection coating for the scan lenses is important. Most manufacturers have standard designs with maximum transmission and minimum reflection and absorption for the wavelength specified by the user. A dual coating may be necessary as well to enable visible transmission through the lens, because scanning systems that use these types of lenses often feature through-the-lens vision systems for checking the quality of the work.
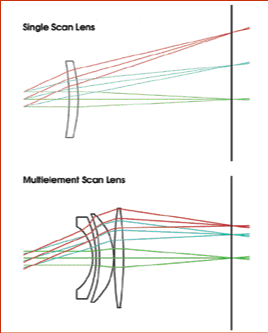
Figure 4. Where precision marking is required, a multielement scan lens replaces a single scan lens because it minimizes spot distortion and drill angle by accepting the laser beam from different angles of input and focusing it perpendicularly.
Band-selective resonator optics
Although positioning the laser beam is of primary importance in applications such as microvia drilling, the wavelength of the laser beam also can affect materials processing efficiencies. This is especially true when using CO2 lasers.
Most CO2 lasers operate at 10.6 µm. This is suitable for cutting steel and certain other materials, but applications such as plastics processing require a different wavelength. Band-selective resonator optics effectively "lock" the CO2 laser to a specific wavelength for specialized industrial applications, such as 9.3 µm for microvia drilling and plastics engraving.
Harmonious optics
Working in harmony, beam expanders, galvo mirrors and scan lenses precisely guide a laser beam to a work surface, enabling users to mark, engrave and drill holes within milliseconds. Advances in optics and innovations such as band-selective resonator optics will ensure that these industrial laser applications continue to grow.