Applying Ancient Technologies to Modern Photonics
Jamie Knapp, Newport Corp. – Corion Optical Filters
One source rarely considered when addressing photonics issues is our ancestors: Great technological discoveries from many millennia ago may lead to modern day solutions.
A recent example of this involves optimizing gold-based infrared reflectors commonly employed for defense and aerospace instrumentation, imaging optics and Fourier transform infrared (FTIR) spectroscopy (environmental monitoring).1 In the latter case, light is transmitted through the volume of air to be analyzed. The light is reflected – via gold retroreflector arrays – back into appropriate analysis instrumentation, where optical absorption is used to evaluate pollutant content. Open-path environmental FTIR spectroscopy is used to monitor CO, CO2, NO and SO2 (absorption peaks are in the range of 1580 to 15,000 nm). HF, HCl, H2S, NH3, CH4, CO2, HCN, C2H4 and C2H2 are measured using tunable diode laser spectroscopy in the 1300- to 1700-nm range.
In the open environment, such mirrors inevitably become soiled and dulled. When cleaned, the soft gold surfaces are damaged. Current conventional gold mirrors, which are deposited upon polished substrates, are therefore oftentimes produced with protective overcoats – common thermal and electron beam-deposited films include silicon monoxide, zinc sulfide and silicon. Such technologies mandate the use of elevated temperatures; e.g., approximately 300 °C. For conventional metal mirrors, these manufacturing techniques are routine.2
An alternative to costly front-surface mirrors is replication, a well-established technology employed to produce high-quality mirrors at a significantly lower cost. These mirrors, however, consist of a critical epoxy layer that is sensitive to elevated temperatures above approximately 105 °C. Standard techniques normally employed to create “hardened” gold mirrors therefore cannot be used.
Unless deposited at elevated temperatures, many protective thin films exhibit poor adherence to the underlying gold and have a porous, columnar micromorphology, limiting their protective properties.3 There is a critical need, therefore, to develop hard, durable, replicated gold mirrors using means that do not involve the elevation of temperature. Such a product must maintain an adequate infrared reflectivity, particularly in the critical 1300- to 1700-nm and 1580- to 15,000-nm ranges.
To address this, one may turn to the Ancients. Mating metallurgical discoveries of almost 2600 years ago, together with state-of-the-art low-temperature thin-film deposition methods, allows for development of the desired hardened replicated infrared reflectors.
History
Introduced almost three millennia ago, coins are an integral part of daily life. From generation to generation, rulers, cities and states have issued a countless number of coins.
Around 670 BC, the ancient Greeks of Ionia and Lydia – now located in modern-day western Turkey – experimented with producing standardized preweighed lumps of electrum, a natural gold and silver alloy found in local river beds.4 To minimize counterfeiting, the lumps were struck with a chisel to expose their inner core; counterfeits were produced by metal-plating electrum onto low-value bronze.
Unfortunately, this did not slow the innovative counterfeiters who managed to produce bronze-cored clones. The first true coin, a piece of metal certified to be of a guaranteed designated monetary value by a recognized governmental authority, was created by King Alyattes of Lydia in 610 BC. The king’s official emblem of the lion acted as a deterrent to counterfeiters (Figure 1).
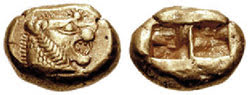
Figure 1. A typical electrum trite from circa 610 BC bears the emblem of King Alyattes of Lydia. Images courtesy of Newport Corp.
Electrum, however, suffers from a variable alloy composition – gold content varied from 45 to 55 percent. Exchange values from coin to coin, therefore, could vary. To address this, under the rule of the legendary King Croesus of Lydia, circa 570 BC, metallurgists developed a means to divide and purify the gold and silver from raw electrum.5 Separate gold and silver coins – 99 percent purity – formed the first “bi-metallic” currency.
The resultant wealth of this Greek region became too much of a temptation to the neighboring ancient Persians. In 540 BC, Croesus was defeated and his empire destroyed. Afterward, King Darius of Persia began striking his new coin (Figure 2).
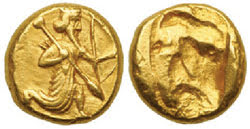
Figure 2. The gold daric, named for King Darius of Persia, was created after the defeat of Croesus in 540 BC. This coin bears the image of the king and was fashioned from a wear-resistant gold alloy.
Known as a gold “daric,” this important coin featured the king’s image, so it was vital that it not suffer wear, as was common in Croesus’ previous pure-gold issues. Persian metallurgists created an alloy that not only maintained the desired visual brilliance of the coin but that significantly added to its hardness and wear resistance.
X-ray fluorescence
To unlock the secrets of Darius’ coins, the nondestructive method of energy-dispersive x-ray fluorescence spectrometry (XRF) was employed. With this technique, the sample is irradiated with x-rays, and re-emitted x-rays have wavelengths that are characteristic of the sample composition.6 Using XRF, the average metallic composition of the Persian daric was found to be 95.1 percent gold, 2.8 percent copper and 2.1 percent silver.
Alloy-replicated mirrors
Replicated mirrors were produced using Darius’ alloy. The intent was to comparatively characterize the surface hardness and wear resistance of this unique alloy against virgin gold and to spectrophotometrically measure its infrared reflectivity. If improved hardness was evident, then the alloy might be an ideal candidate for further surface hardening using a room-temperature deposited protective film.
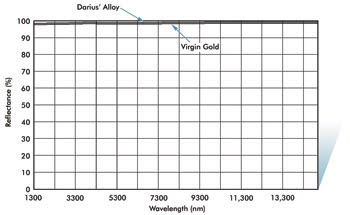
Figure 3.
For comparative hardness measurements, the moderate abrasion methods per MIL-M-13508C were used by abrading dry cheesecloth across a mirror’s surfaces using a 1-lb force. Virgin gold suffered visual damage after one test stroke; Darius’ alloy survived eight to 12 strokes.
Reflectivity was measured on PerkinElmer Lambda 950 UV/VIS and Lambda 983 infrared spectrophotometers. In the 1300- to 15,000-nm region, alloy mirrors were basically indistinguishable from virgin gold (>99 percent reflectivity).
These alloy-replicated mirrors therefore were excellent candidates for further surface modifications.
Reactive ion plating deposition
Established as a means to produce high-quality optical filters, Newport’s Stabilife Reactive Low Voltage Ion Plating (RLVIP) process was used to deposit hard adherent silicon protective films upon the alloy mirror’s surfaces.7 The process produces optical thin films possessing the physical density, amorphous microstructure and optical properties approaching those of bulk materials. These homogeneous films are free of the physical pores and columnar structure typically seen in coatings created by other means. Of prime importance is that deposition takes place at room temperature.8
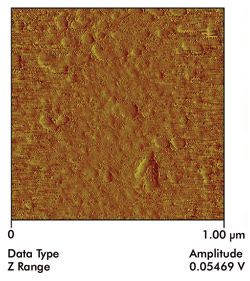
Figure 4. This AFM image shows the surface micromorphology of a 2-μm-thick silicon film deposited by conventional thermal evaporation at room temperature.
Using atomic force microscopy9 (AFM), Figure 4 shows the surface micromorphology of a 2-µm-thick silicon film deposited by conventional thermal evaporation at room temperature. The resultant film morphology exhibits a rough, poorly nucleated, nonadherent columnar porous film.
Figure 5 is an AFM evaluation of a 2-µm silicon film created at room temperature by the reactive ion plating. In this case, the film is fully adherent, smooth and featureless, indicative of a fully densified bulklike thin-film structure.
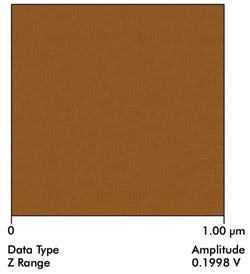
Figure 5. An AFM evaluation of a 2-μm silicon film was created at room temperature by Newport’s patented Reactive Low Voltage Ion Plating process.
Protected gold alloy-replicated mirrors
Replicated mirrors produced with Darius’ alloy subsequently were coated with elemental silicon at room temperature using RLVIP. A film thickness of 70 nm was employed to tune the optimal spectral performance for the desired 1580- to 15,000-nm spectral region. A silicon film thickness of 160 nm was used for creating mirrors for the alternative 1300- to 1700-nm band. The resultant measured reflectances demonstrate that these surface-modified replicated mirrors are indeed well suited to critical infrared instrumentation applications (Figures 6 and 7).
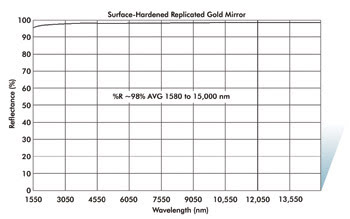
Figure 6.
For a comparative measurement of surface hardness and abrasion resistance, the moderate abrasion methods as described in MIL-M-13508C were re-employed. In both of the above cases, RLVIP-protected replicated gold alloy mirrors survived beyond 350 strokes without visible evidence of any surface abrasions.
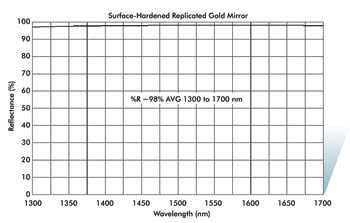
Figure 7.
Learning from the past
Many ancient civilizations have contributed to our current modern scientific understanding. Countless groundbreaking methods were created many millennia ago, many of which still offer rare insights when developing new technologies.
In this current case, the successes of ancient metallurgists became key in developing an improved cost-effective replicated gold-based infrared reflector. The ancient Greeks and Persians were not merely scientific theoreticians; a multitude of discoveries were transformed into practical applications.
Meet the author
Jamie Knapp is director of engineering at Newport Corp. – Corion Optical Filters in Franklin, Mass.; e-mail: [email protected].
References
1. T.R. Minnich and R.L. Scotto. “Use of open-path FTIR spectroscopy to address air monitoring needs during site remediations.” Remediation Journal, Vol. 9, No. 3, pp. 79-92.
2. L. Maissel and R. Glang. Handbook of Thin Film Technology (1970). McGraw-Hill.
3. H.K. Pulker. Coatings on Glass (1984). Elsevier. 1984.
4. C.M. Kraay. Archaic and Classical Greek Coins (1976). Methuen Young Books.
5. A. Ramage and P. Craddock. King Croesus’ Gold: Excavations at Sardis and the History of Gold Refining, Harvard University Art Museums, 2000.
6. B. Beckhoff, et.al. Handbook of Practical X-Ray Fluorescence Analysis (2006). Springer.
7. J. Knapp, C. Burke and R. Filippi (1994). “Environmentally stable optical filters for use in fluorescence chemical sensing instrumentation.” “Optical Sensors for Environmental and Chemical Process Monitoring.” Proceedings SPIE, Vol. 2367
8. K.H. Gunther, B. Loo, D. Burns, J. Edgell, D. Windham and K.H. Muller (1989). “Micro structure analysis of thin films deposited by reactive evaporation and by reactive ion-plating.” Journal Vac. Sci. Technol., A7.
9. T. Bieler, Department of Chemical Engineering and Material Science, Michigan State University, 2008.