Edith Innerhofer, Felix Brunner, Sergio Vincenzo Marchese, Dr. Rüdiger Paschotta and Prof. Dr. Ursula Keller, ETH Zürich
Despite being considerably more expensive than TV sets, liquid crystal display projectors are finding their way into many homes. Their large projection area and deep color saturation generate something close to a cinematic experience in the living room. To keep their customers, movie theaters will have to provide an even better show. Although nearly all theaters currently rely on old-fashioned film projectors, digital laser projectors can provide a superior moviegoing experience because they generate very sharp and flicker-free images with much brighter colors. Moreover, they offer the advantages of other digital media: compact storage, fast distribution and flexible handling.
The technologies for projectors as well as for management of digital media already exist and work well. But lasers capable of powering these projectors have been the missing link. The lasers would require attributes such as a compact form factor, high efficiency, stability and robustness, all without excessive complexity. At ETH Zürich, an important step is being taken in this direction: the development of a very powerful RGB (red-green-blue) laser source that is simpler than previous devices.
A seemingly straightforward approach for an RGB system is based on separate lasers for red, green and blue outputs. But a more promising approach to an efficient and commercially viable RGB source is based on a single infrared laser that typically operates in the 1-μm region and is combined with several nonlinear conversion stages to convert the invisible laser light into red, green and blue light.
The first important building block of our system was provided by the development of a novel class of infrared lasers that generate ultrashort pulses with durations of less than 1 ps and very high average and peak powers. In 2000, our group demonstrated passive mode locking of a thin-disk Yb:YAG laser, leading to as much as 16 W of average power at 1030 nm in subpicosecond pulses.1 This laser not only generated much higher powers than previous models, but also was the first femtosecond laser based on a power-scalable design. Power scalability means that there is a way to scale up the output power without encountering fundamental challenges.
One such challenge is thermal lensing in the gain medium, which normally gets worse for higher powers and which eventually limits the output of the laser. The thin-disk laser concept, however, allows power scaling without increasing the influence of thermal lensing — at least as long as thermal stress in the disk does not become too strong. This can be achieved with a sufficiently thin gain disk.
Good pumping efficiency can be maintained with special pump optics that effect multiple passes of the pump radiation through the disk. The experimental demonstration of significantly enhanced power came in 2003 when we increased the power level from 16 to 60 W, while maintaining the short pulse duration and excellent pulse quality.2 More recently, the laser’s output has been increased to 80 W (Figure 1).
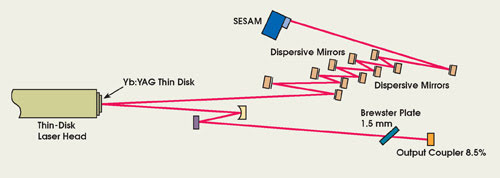
Figure 1. In this experimental setup of the Yb:YAG thin-disk laser, which includes the laser head, a number of dispersive dielectric mirrors and a semiconductor saturable absorber mirror (SESAM) as passive mode-locker, the laser generated an average power of 80 W in 705-fs pulses.
A more traditional approach for an RGB pump source is to use a neodymium-based seed laser with just a few watts of output power and to amplify this output with one or several amplifier stages. Although this source delivers as much as 42 W of average power in short pulses, it requires a more complex setup with four sequential amplifier stages.3 And because of the limited gain bandwidth of neodymium-doped crystals, the pulses are about an order of magnitude longer than those from the thin-disk Yb:YAG laser.
The importance of short pulses in an RGB source has only recently been understood. With subpicosecond pulses from the laser, the peak powers are so high that only weak focusing into the nonlinear crystals is needed. This makes it possible to employ critical phase matching by angle-tuning the crystal while it remains at or near room temperature. In contrast, previous laser systems did not reach high enough peak powers and, therefore, required noncritical phase matching to accommodate the sharply focused beams.
In noncritical phase matching, the crystal must be heated to its phase-matching temperature and held there in a temperature-stabilized oven. Obviously, the need for several ovens makes the whole system considerably more complex and expensive, and introduces a significant warm-up time for the crystals.
There are, in principle, many ways to arrange nonlinear conversion stages for the generation of red, green and blue outputs.4 The methods differ not only in the number of crystals and intermediate wavelengths involved, but also in the materials of the required crystals, the phase-matching schemes and the required optical resonators. Factors other than power efficiency strongly influence the suitability of a conversion scheme for commercial applications.
Ideally, one wants a system that relies only on robust crystals that are easily available and that are of good quality and low in cost. The system described here contains four crystals of LBO (LiB3O5), all of which can be operated at room temperature, and a single crystal of periodically poled stoichiometric lithium tantalate (PPSLT), which currently requires a temperature-stabilized oven. There is the hope that further modification of this material (with MgO doping) also will make possible room temperature operation, so that the RGB system will not need even a single crystal oven.5
Another important consideration is that a synchronously pumped parametric oscillator is often needed, and the fact that the parametric oscillator’s length must match that of the pump laser precisely makes the alignment more difficult and the setup less compact. In most cases, particularly for femtosecond pulses, the cavity length must be actively controlled, adding to the complexity of the system.
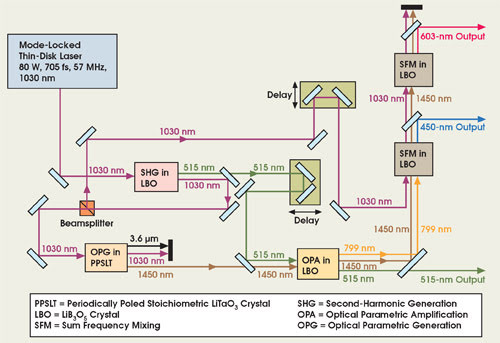
Figure 2. In this experimental setup of the RGB source, all beams are collinear in the nonlinear crystals, although for clarity they are shown with some spatial separation.
Parametric generator
The ETH system does not need such a cavity (Figure 2). Instead, a parametric generator based on the PPSLT crystal is used. To avoid problems with crystal damage and certain beam-quality issues, we operate the parametric generator at a moderate average output power level of about 1.6 W.6 A high-power parametric amplifier based on an LBO crystal boosts the power at 1450 nm while generating an additional idler wave with 799 nm. The amplifier is pumped with green light, which is obtained by frequency doubling the laser output in another LBO crystal. The remaining green light can be used for the display, while the red and blue outputs are obtained in two sum frequency stages, based on LBO, which mix the signal and idler outputs with the remaining pump light from the frequency doubler.
Because of the multiple usage of various beams, the overall efficiency of the conversion system is rather high. From 80 W of laser power, we currently obtain a total of 41 W in the visible outputs (Figure 3). Our RGB source’s total conversion of infrared (1030 nm) to visible is 51 percent. For the generation of D65 white light (ISO10526/CIES005/E), the red color currently limits the total output power. The color-balanced output would be 24.9 W of white light, which corresponds to 7840 lumens (calculated with 8 W of red, 6.4 W of blue and 10.5 W of green).
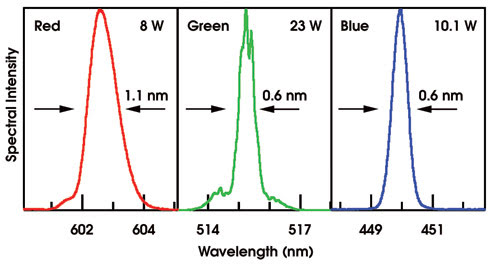
Figure 3. Optical spectra of the 603-nm red, 515-nm green and 450-nm blue output of the RGB system measure 8, 23 and 10.1 W, respectively.
By further fine-tuning the system, we hope to improve the power balance toward more red and blue. Also, it is possible to change the wavelengths of the red and blue light simply by changing the poling period of the PPSLT crystal. For example, a signal wave of 1563 nm instead of 1450 nm could be generated, resulting in a blue wavelength of 440 nm and a red wavelength of 620 nm, which would enable better color saturation of the display.
The beam quality is close to the diffraction limit for the red and blue beams, while the divergence of the green beam is approximately 1.9 times higher than the diffraction limit. However, the high power in the green would allow for some power loss caused by any spatial filtering that is needed for the projector. Also note that not all projectors require perfect beam quality of the laser source.
Besides unprecedented output,7 this RGB laser source has several attractive properties. A single laser oscillator provides power for the nonlinear conversion stages. The system needs no laser amplification stages nor any synchronized optical cavities. It is based on critically phase-matched crystals operated at room temperature, except for the first optical parametric generation stage, where we use the crystal at an elevated temperature. We expect that it will also become possible to operate this first stage at room temperature with improved materials such as magnesium-doped PPSLT.5
This laser system represents a big step toward a practical, high-power laser projection display for theaters. Another potential application is in-flight simulators, where a realistic, high-quality projection system is required.
References
1. J. Aus der Au, G.J. Spühler, T. Südmeyer, R. Paschotta, R. Hövel, M. Moser, S. Erhard, M. Karszewski, A. Giesen and U. Keller (2000). 16.2 W average power from a diode-pumped femtosecond Yb:YAG thin disk laser. OPT. LETT. 25, 859.
2. E. Innerhofer, T. Südmeyer, F. Brunner, R. Häring, A. Aschwanden, R. Paschotta, U. Keller, C. Hönninger and M. Kumkar (2003). 60 W average power in 810-fs pulses from a thin-disk Yb:YAG laser. OPT. LETT. 28, 367.
3. R. Wallenstein (2001). Advanced solid state sources for high power visible light generation. Technical Digest of Conference on Lasers and Electro-Optics, paper CThC3.
4. R. Wallenstein, US Patent No. US005828424, “Process and Apparatus for Generation…” (1998).
5. K. Kitamura, N.A. Yu, M. Nakamura and S. Kurimura (2004). 2×2×35L mm3 rod-type periodically poled 1.0 mol% MgO-doped stoichiometric LiTaO3 for optical parametric oscillation. Technical Digest of Advanced Solid State Photonics, paper PD10.
6. G. Arisholm, T. Südmeyer and R. Paschotta (2004). Limits to the power scalability of high-gain optical parametric oscillators and amplifiers. J. OPT. SOC. AM. B 21, 578.
7. F. Brunner, E. Innerhofer, S.V. Marchese, T. Südmeyer, R. Paschotta, T. Usami, H. Ito, S. Kurimura, K. Kitamura, G. Arisholm and U. Keller (2004). Powerful RGB laser source pumped with a mode-locked thin disk laser. Accepted for publication in OPT. LETT.
Meet the authors
Edith Innerhofer, Felix Brunner and Sergio Vincenzo Marchese are research assistants at the Swiss Federal Institute of Technology (ETH) in Zurich, Switzerland. They have diplomas in physics from the institute.
Rüdiger Paschotta is a research group leader at ETH Zürich. He has a PhD in physics from the University of Konstanz and received his habilitation in experimental physics from the institute at ETH.
Ursula Keller is a full professor of experimental physics and head of the institute, where she received a diploma in physics. She earned her MS and PhD in applied physics from Stanford University. She also is a director and scientific adviser to Time-Bandwidth Products Inc.
Concept of a Thin-Disk Laser
The gain medium of a thin-disk laser head, as invented by the group of Adolf Giesen at the University of Stuttgart, Germany, is a very thin disk, typically 100 μm thick, that is usually made of Yb:YAG.2 One end face of the disk is coated for high reflectivity and mounted on a heat sink.
Because the disk’s pump and laser beam diameters are much larger than its thickness, we have a quasi-one-dimensional heat flow into the heat sink and, therefore, only weak thermal lenses and aberrations. The same applies to the semiconductor saturable absorber mirror, where the absorber thickness is smaller than the beam diameter. The concept of a mode-locked thin-disk laser, therefore, is power scalable. The absorption length of the gain material (typically a few millimeters) is much longer than the disk thickness, so the pump absorption in a single pass is weak.
Efficient pump absorption can be achieved in a compact multipass arrangement of pump radiation (see figure). In this configuration, we have 16 passes through the disk. This arrangement is realized with a parabolic mirror and a number of roof prisms. With the thin-disk laser concept, up to ~100 W in a diffraction-limited continuous-wave output has been demonstrated, and the 80 W in 705-fs pulses directly out of a mode-locked laser oscillator are, to our knowledge, the state of the art.