Andrew Held and Arnd Krueger
The high peak powers, picosecond pulse durations and high repetition rates of mode-locked Nd:YVO4 lasers provide an edge in ultrafast research, materials processing and flow cytometry.
Flexibility may be the top selling point of mode-locked, diode-pumped Nd:YVO4 lasers. Their
80-MHz repetition rates allow them to compete in applications typically reserved
for CW sources such as argon-ion lasers. Their combination of high peak powers and
picosecond pulse durations enables precision machining by incising finer and thinner
cuts with almost no peripheral thermal damage. Their high peak powers also support
efficient harmonic generation, providing several watts of power in the green, the
UV and the deep-UV.
These Nd:YVO4 lasers both complement and compete
with conventional lasers. In micromachining applications, for example, mode-locked
devices offer an operating regime complementary to high-speed Q-switched lasers
in terms of repetition rate and peak power.
Ultimately, the key to mating these
lasers with the right applications lies in developing an understanding of how they
work.
How they work
In a mode-locked laser, the phases of the longitudinal
cavity modes are locked together. In the time domain, this generates one intense
pulse that circulates around the cavity, producing pulsed output. Mode-locking can
be active or passive in nature.
In one such laser — Spectra-Physics’
Vanguard — engineers replaced the rear high reflector with a passive component,
a saturable Bragg reflector. Originally developed and patented by Lucent Technologies
Inc. of Murray Hill, N.J., this highly reflective cavity mirror also acts as a passive
mode-locking element. Its outer layer contains a quantum well of InGaAs thick enough
to absorb 1064-nm laser radiation.
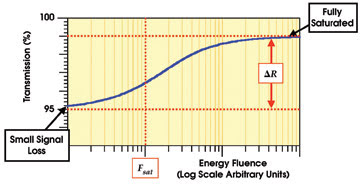
In a saturable Bragg reflector, the absorption saturates at higher intensities, thereby increasing its reflectance. End users can tailor both the absorption and the saturation fluence for individual applications.
When the laser operates in CW mode
at low fluence, its reflector introduces significant cavity loss. The high peak
powers that are characteristic of mode-locked operation, however, bleach the reflector
and reduce the loss. Because a laser always seeks to operate at the highest value
of gain minus loss, the saturable Bragg reflector automatically produces mode-locking
that does not suffer from dropouts, unlike earlier passive techniques.
The time it takes for a mode-locked
pulse to circulate around the cavity determines a mode-locked laser’s repetition
rate. Thus, longer cavities deliver lower repetition rates and vice versa. Research
has shown that 80 MHz is the optimum repetition rate for most applications. A lower
rate would not satisfy the demands of CW and quasi-CW applications. For a given
average power, a faster repetition rate would reduce the peak power of the individual
pulses, which could limit the advantage of these lasers for materials processing
and efficient harmonic generation.
In a mode-locked laser, the shortest
possible pulse is inversely proportional to the spectral bandwidth of the output.
In an Nd:YVO4 laser, the typical bandwidth of ~180 pm (~50 GHz) translates
to a minimum pulse width of 6 ps. In practice, these new lasers can deliver infrared
pulse widths of approximately 12 ps.
The properties of Nd:YVO4 make it possible
to extract relatively high power from a single laser rod, which simplifies the cavity
design. The end-pumped rod has a single diode to maximize mode quality and TEM00
power. This configuration delivers 6 W at 1064 nm, 2 W at 532 nm using an extracavity
doubler module, and more than 350 mW at 355 nm with a tripler module.
A higher-power version uses a simple,
double-pass amplifier, wherein the laser pulse passes twice through a second Nd:YVO4
rod that is pumped by diodes at both ends. This produces more than 20 W at 1064
nm, 10 W at 532 nm and 4 W at 355 nm. Because of the short pulse duration, the peak
power is several kilowatts, making this laser suitable for materials processing
applications.
Diode-pumped Nd:YVO4 lasers are also
compact: Although an 80-MHz repetition rate requires a cavity length of 1.8 m, these
lasers have a folded cavity that reduces the head size. They require no external
cooling water, operate from conventional line voltage and do not need a three-phase
power installation. Because they rely on diode-pumping and permanently aligned components,
diode lifetimes exceed 10,000 hours. Also, because the pump diodes are produced
as fiber-coupled modules and are located in the power supply, no optical realignment
is necessary during diode replacement.
For micromachining, mode-locked lasers
offer an operating regime complementary to high-speed Q-switched lasers in terms
of repetition rate and peak power. In a performance comparison reported at the October
ICALEO conference in Scottsdale, Ariz., both a mode-locked UV laser and a Q-switched
laser produced 4 W of 355-nm UV output. However, the mode-locked device delivered
repetition rates of 80 MHz and pulse widths of 10 ps, whereas the Q-switched laser
operated at 30 kHz with pulse widths of 35 ns.
In the case of metal (copper) samples,
the Q-switched laser proved more effective for drilling and scribing because of
its higher energy per pulse. However, in the case of several nonmetallic samples,
such as epoxy glass and thin films of polyimide, the mode-locked laser delivered
higher cutting speeds and produced more finely detailed cuts. Furthermore, the thinner
the film, the bigger the maximum speed difference between the two laser types.
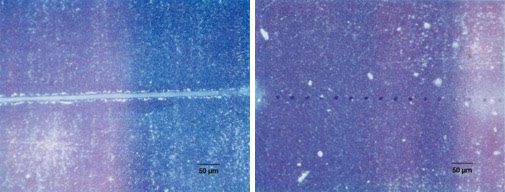
Cuts in epoxy glass made by high-repetition-rate, mode-locked Nd:YVO4 UV lasers (top) are finer and smoother compared with cuts made by fast Q-switched lasers of the same average power (bottom). The devices scribed samples at a scan speed of 1000 mm/s.
In 50-μm polyimide, for example,
the mode-locked laser was 7.5 times faster and produced much thinner cuts than the
Q-switched laser. In addition, the high repetition rate of the mode-locked device
produced smooth cuts, with no evidence of individual pulses.
Flow cytometry
One application for CW UV laser radiation is flow
cytometry, in which the source excites multiple fluorophores that emit in different
characteristic spectral regions. In this application, prediluted cells pass a focused
laser beam one at a time in the form of a stream or tiny droplets. The system detects
the fluorescence using a series of photodetectors, each of which features its own
spectral filter. This allows counting or sorting of cells using an electric field
to deflect the cells according to their fluorescence profile.

In flow cytometry, fluorescently labeled cells flow past a focused laser beam. Although the flow rate is high (50,000 cells per second), it is still very slow compared with the time scale of a mode-locked, 80-MHz laser.
In a high-performance flow cytometer,
the typical flow rate is approximately 50,000 cells per second. In that time frame,
an 80-MHz, mode-locked laser seems to be CW. Recent research by scientists at the
Laboratory for Cell Analysis at the University of California, San Francisco, and
colleagues at Becton Dickinson BioSciences in San Jose, Calif., confirmed the suitability
of these lasers in a project using a 355-nm, oscillator-only device that produced
350 mW.
A key question answered by this study
was whether the high peak power would kill or damage the sorted cells. In fact,
with its power attenuated to below 260 mW, the laser appeared to have no effect
on cell viability.
As a result, the scientists concluded
that this laser works well in research and clinical laboratory applications of flow
cytometry and that it could replace UV argon-ion lasers in many cases.
Circuit boards
Direct imaging of printed circuit boards also
requires CW or quasi-CW UV laser radiation. The traditional board fabrication process
relies on light to polymerize a photoresist, followed by a wet-chemistry photoetch
process and copper plating. Engineers usually create the design for exposing the
photoresist as a negative image, but this introduces several limitations, especially
with the trend to push line densities ever tighter to meet the demand for miniaturization
and increased functionality in consumer products.
First, the plates may distort slightly
as a function of temperature and humidity. In fact, most circuit board manufacturers
keep several plates for different ambient conditions. Second, layer-to-layer registration
becomes increasingly important and difficult at high line densities, requiring the
use of oversize copper pads on each layer to ensure that the vias form good electrical
connections between the layers. In addition, the plates are not robust, having a
finite lifetime. Fixed artwork also limits flexibility and increases the cost of
short, prototype production runs.
One solution is to create the desired
pattern in the photoresist with a scanning UV laser beam. Ion lasers are not good
choices for this application because of their high power and water consumption and
because of the limited lifetime of their plasma tubes. Q-switched solid-state UV
lasers also will not work because their pulse rates could potentially limit process
throughput. At the high scan speeds delivered by the polygon mirrors, the scanned
laser beam would produce a broken line rather than the desired continuous line.
Thus, the high-repetition-rate, mode-locked
quasi-CW laser becomes a good choice to meet the needs of this growing application.
Moreover, with up to 4 W at 355 nm from an oscillator/amplifier model, beta testing
in this application indicates that the output power is sufficient to support economically
attractive throughput rates.
What’s ahead
Mode-locked, diode-pumped lasers also offer potential
benefits to other applications. For example, the research community has a large
installed base of synchronously pumped dye lasers supported by a generation of failing
lamp-pumped, ultrafast Nd:YAG lasers. These lasers perform multiphoton spectroscopy
and time-resolved photochemistry at “difficult” wavelengths. The Nd:YVO4
systems can serve as a replacement pump source for these dye lasers — an application
that represents a sizable potential market in the near term. In fact, a 2-W green
model has a cavity length optimized to deliver a choice of 76- or 80-MHz outputs,
the typical repetition rates for commercial ultrafast dye lasers.
Meet the authors
Andrew Held is marketing manager for the diode-pumped
solid-state Q-switched laser division at Spectra-Physics in Mountain View, Calif.
Arnd Krueger is marketing manager for
the company’s continuous-wave and ultrafast laser group.