These nearly invisible particles are changing the way we approach light conversion, resulting in more efficient, more colorful, and brighter displays.
PETER PALOMAKI, PALOMAKI CONSULTING
Quantum dots. QDs. QLED.
The presence of quantum dots (QDs) in consumer displays has been on the rise since Sony introduced QD Vision’s edge optic in its 2013 Triluminos TV series. Samsung introduced its super ultrahigh-definition QLED TV two years later, accompanied by a strong marketing campaign for QLED, which has since driven interest from consumers and display makers alike. With improved color and energy efficiency, nearly every major display manufacturer is now incorporating QDs into their high-end displays.
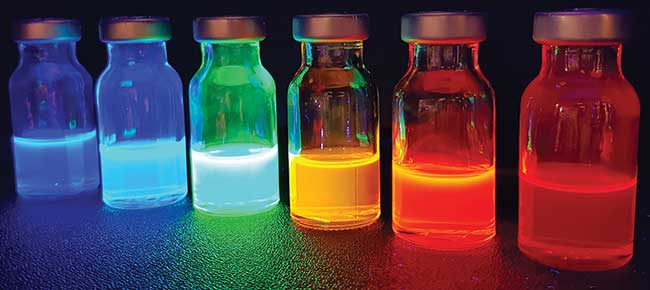
Vials of quantum dots, with their unique emission properties revealed. Courtesy of Quantum Solutions.
QDs are inorganic semiconductor nanoparticles — technically, nanocrystals — that are about 10 nm in diameter, 10,000× smaller than the diameter of a human hair (Figure 1). QDs are very good at absorbing high-energy blue light and reemitting that light at longer wavelengths, such as green and red. The specific wavelengths that QDs absorb and reemit depend on their composition and size.
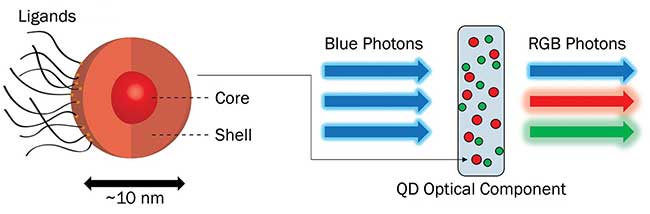
Figure 1. Composition and function of a quantum dot (QD). Courtesy of Nanosys.
What is unique about QDs compared to other down-converting materials such as phosphors is their combination of small size, tunability, efficiency, and narrow emission profile. In QD-enabled displays (sometimes called QLED technology), there are both red and green QDs typically mixed together in a polymer to create high-quality red, green, and blue light when stimulated by a blue LED.
Creating colors, enhancing efficiency
Nearly all modern LCD displays rely on red, green, and blue primary colors to generate the full-color image we see every day on our phone, TV, and computer displays. Photons are first generated by a blue LED, then down-converted to various visible wavelengths by a phosphor — commonly, yttrium aluminum garnet (YAG). This broadband white light passes through color filters to isolate only red, green, and blue photons. Those that are in between the RGB primaries are blocked by the color filter, resulting in a loss of efficiency (Figure 2, top row).
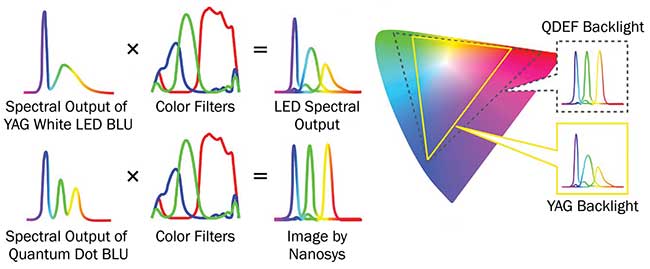
Figure 2. Spectral output of blue LED + YAG phosphor (top row) compared to blue LED + QDs (bottom row). Spectra generated by the phosphor/QDs are then passed through a color filter to generate the final LCD spectrum. The QD down-converter approach produces a larger color gamut with less wasted light.
Compared to YAG phosphor, QDs have narrow emission with excellent control of the wavelength, such that the exact QD color can be preselected based on size and composition. The result: Most of the generated photons pass through the color filters instead of being blocked, which means more efficient use of the photons (Figure 2, bottom row). A big selling feature for QD-enabled displays is the increased color gamut and enhanced efficiency. The increased color allows for a more realistic rendering of lifelike colors, and increased efficiency means reduced energy consumption and fewer LEDs required in the display. In addition, the use of QDs opens up additional design options for displays to more effectively harness the efficiency and narrow emission of these materials.
There is always a trade-off between efficiency and brightness in displays. Display manufacturers, and consumers to some degree, can choose between a more efficient display with fewer LEDs and lower drive current, or a higher brightness display with more LEDs and higher drive current. Depending on how they are integrated, QDs enable a more efficient device and improved brightness. The type of quantum dot also has a big impact on the display properties.
Types of quantum dots
Today’s scientists can create dozens of different QD materials, but only a small fraction of those are suitable for use in displays. To be considered, the QDs must absorb blue photons, efficiently convert blue to narrow red and green photons, be stable for tens of thousands of hours, and be scalable yet inexpensive enough to meet customer demand now and in the future. The two major QD technologies that meet these criteria are those based on cadmium selenide (C
dSe) and indium phosphide (InP). (Table 1 summarizes the properties of these two materials and a common phosphor, YAG.)
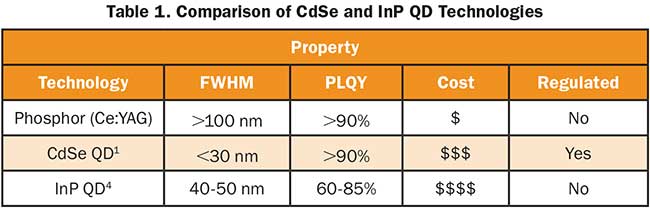
Table 1. FWHM: Full width half maximum; PLQY: photoluminescent quantum yield; $ to $$$$: least to most expensive.
The two most important metrics used in evaluating down-converters for displays are the full width at half maximum (FWHM, a measurement of emission peak width) and photoluminescent quantum yield (PLQY, the efficiency with which a blue photon is converted to another color). The narrower the peak width, the better the color quality. The higher the quantum yield, the more efficient and brighter the display. CdSe QDs are the winner in both categories. However, InP has seen better penetration in the display market because of one major factor: the absence of the regulated element cadmium.
CdSe QDs were the first material implemented in displays mainly because they have had more time to mature; CdSe was one of the materials originally studied for QDs in the early 1990s1.
There has been staggering progress in optimization of their optical properties, methodologies for synthesis, and scalability in the past few decades. CdSe QDs were an obvious choice for development because of their efficient and narrow peaks. However, regulatory bodies have imposed limits on the amount of cadmium that can be incorporated in consumer electronics.
One of the main regulations on cadmium comes from the European Commission’s restriction of hazardous waste, which allows no more than 100 ppm of cadmium to be present in any component of a consumer electronic device. Because of the enhanced efficiency of displays using CdSe QDs, there is currently an exemption in place that allows cadmium levels above this limit to be used in displays. This exemption was recently extended to 2019, pending any other changes2.
InP QDs were first synthesized3 in 1994. However, because of difficulties in their preparation and less-than-ideal optical properties, only recently have academic groups and companies focused on improving the properties of InP to a commercially competitive status4. Current optical properties (FWHM and PLQY) for InP still lag behind typical properties of CdSe, but the gap is narrowing with improvements in the fundamental understanding of materials and improved manufacturing methods.
There are other QD types on the horizon that look promising, but further development is required before they can compete with CdSe and InP. Perovskite (the same material being pursued for high-efficiency solar cells) QDs can have very narrow emission in the visible range, making them attractive for pushing closer to wide color gamut Rec. 2020 specifications5. However, these materials contain the regulated compound lead and currently have insufficient long-term stability that is required for consumer displays. While the type of QD is important, the way in which the QDs are implemented can result in even greater impact and benefits for consumer displays.
QDs in displays today
To date, only two methods of QD implementation have been available in displays: film and edge optic. In both methods, blue LEDs are used as the illumination source, and QDs are dispersed in a polymer that is protected from atmospheric degradation by external packaging. Both provide the important benefits of enhanced efficiency and brightness and an improved color gamut. The differences lie in how they are implemented into the display backlight unit.
Film: Red and green QDs are homogeneously dispersed in a polymer film, which is then placed into the backlight unit along with several other optical films. Light conversion happens over a large area and can be illuminated by LEDs on either the edge or back of the display. Typically, the QD-polymer composites are sandwiched between two expensive barrier films that prevent atmosphere from reaching the QDs and degrading their emissive properties.
Edge optic: QDs are dispersed in a polymer inside of a glass tube on top of the strip of LEDs at the edge of the display. This method uses far fewer QDs than the film option but can only be used with edge-lit displays. Since this method is closer to LEDs, the light flux and temperature is greater and a hermetic glass seal is required to ensure stable operation.
There are many advantages with either implementation strategy, but the main reason film has become the dominate form factor for QDs in displays is the ease of implementation and ability to integrate into nearly any LCD type (curved, edge-lit, backlit, large TV, small tablet).
Film is more expensive than edge and uses more QD material, but the benefits outweigh the drawbacks for most display manufacturers. With the sale of QD Vision to Samsung in 2016, the edge optic became obsolete. All commercial displays that currently incorporate QDs use the film approach. Even though film may be the form factor of choice for now, there are many ways QDs could be integrated into future displays.
Future of QDs in displays
QDs as color filter replacements may be the next big breakthrough for displays. In this approach, red and green QDs are patterned on the subpixel level and act as the color filter and color converter in one step. The blue subpixel would simply pass through the blue light from the LEDs.
If successful, this implementation of QDs could result in dramatically improved efficiency by more than 2× over film implementation and better viewing angle (rivaling OLED), along with the enhanced color that all QD implementations enable6.
However, the challenges to overcome are substantial. One is the redesign of the LCD stack to incorporate the second polarizer in the cell with QDs following. This is a requirement because QDs depolarize light. Subpixels require
polarized light to operate, and if QDs are utilized in the same position as current color filters, the LCD will not work. This alone represents a dramatic change to the LCD production process, which must be driven by the panel makers, not the QD component suppliers. The component makers must be able to create extremely thin films with very high QD concentrations such that they absorb nearly 100 percent of the blue light that strikes each subpixel.
In addition, subpixel-level patterning or printing must be implemented, which means very different process requirements for the QD/polymer composites; some require high-temperature curing steps.
Even if all of these issues can be solved, there are additional challenges in light management: preventing unintentional excitation from blue room light, extracting all photons from QDs that emit in all directions, and reducing or eliminating unintentional crosstalk among neighboring subpixels. Look for this technology to be available in the next few years.
On LEDs and microLED: Red and green QDs are dispersed in the silicone encapsulant on top of the blue LED die. This implementation strategy has the benefit of being incredibly simple — a true drop in substitute for phosphors. If both red and green QDs can be engineered to survive in the high-temperature, high-flux environment of LED chips, this approach could lead to simplified production with less QD usage.
Recent success has been reported by Pacific Light Technologies in Lumileds midpower LEDs to create white lighting with high color quality and high efficacy7,8. The QDs used in these devices are specifically engineered to survive the high-stress environment of LED packages.
Unfortunately, this approach has only worked with CdSe because InP is not stable enough to survive on-chip conditions. It is already available as a lighting product with red CdSe QDs, but further improvements are required to improve green QD performance before a commercial product for displays can be introduced.
Figure 3. Summary of successful current implementation and next-generation implementation methods of QDs in displays. Courtesy of QD Vision, Nanosys, and Lumileds.
MicroLEDs have recently become a hot topic as a next-generation display technology. QDs could play a key role in microLED technology because they are a much smaller size than traditional phosphor particles, which are larger than individual microLEDs. To simplify microLED production and integration into large area displays, blue-only microLEDs could be used with red and green QD down-converters to efficiently generate full-color microLED displays.
Electroluminescent: The ultimate display technology — electroluminescent — is a self-emissive technology that does not rely on inefficient down-conversion of blue photons to red and green photons.
Electroluminescent QD displays would utilize the same QDs in previous strategies, but instead of acting as a down-converter, they are electrically stimulated to emit photons and act as QD LEDs. This technology promises dramatic efficiency improvements since there is no wasted light, and it will lower cost by the elimination of many of the optical components found in a traditional LCD.
This approach would result in enhanced efficiency, brightness, viewing angle, and color, and could potentially open the door to flexible displays.
But don’t expect to see this technology in living rooms any time soon. Electro-luminescent QDs will require major improvements in stability and efficiency before they become a viable option. Additionally, they will require patterning at the subpixel level, but many of the advancements in OLED could be adapted for electroluminescent QD displays.
For now, this approach remains an active research project in both academia and industry. If successful, electroluminescent QDs will truly be a disruptive technology in the display industry.
Based on the rapid adoption of QDs in display technology over the last few years, it seems clear that we are likely to continue to see rapid progress in the incorporation of QD technology into commercial displays.
The current approach of QD films is just the beginning, as there are multiple technological innovations on the horizon that incorporate them into LCD technology.
The upcoming revolution of QD-enabled displays will continue to improve color and efficiency and provide an all-around enhanced viewing experience.
Meet the author
Peter Palomaki, Ph.D., is the owner and chief scientist at Palomaki Consulting. He assists clients with technical projects involving quantum dots, nanofabrication, and nanomaterials that enable next-generation optical technologies; email: [email protected].
References
1. J. Owen and L. Brus (2017). Chemical synthesis and luminescence applications of colloidal semiconductor quantum dots. J Am Chem Soc, Vol. 139, Issue 32, pp. 10939-10943.
2. Commission Delegated Directive for specific exemption 3 from restrictions of hazardous substances (RoHS). http://ec.europa.eu/info/law/better-regulation/initiatives/c-2017-5446_en.
3. O.I. Micic et al. (1994). Synthesis and characterization of InP quantum dots. J Phys Chem, Vol. 98, Issue 19, pp. 4966-4969.
4. S. Tamang et al. (2016). Chemistry of InP nanocrystal syntheses. Chem Mater, Vol. 28, Issue 8, pp. 2491-2506.
5. L. Protesescu et al. (2015). Nanocrystals of cesium lead halide perovskites (CsPbX3, X = Cl, Br, and I): novel optoelectronic materials showing bright emission with wide color gamut. Nano Lett, Vol. 15, Issue 6, pp. 3692-3696.
6. E. Lee et al. (2017). Ambient processing of quantum dot photoresist for emissive displays. SID Symp Dig Tech Paper, Book 2, Session 67, pp. 984-987.
7. K. Shimizu et al. (2017). Toward commercial realization of quantum dot based white light-emitting diodes for general illumination. Photonics Res, Vol. 5, Issue 2, pp. A1-A6.
8. B.D. Mangum et al. (2017). Exploring the bounds of narrow-band quantum dot downconverted LEDs. Photonics Res, Vol. 5, Issue 2, pp. A13-A22.
Quantum Solutions
For decades the term “QLED” was reserved for electroluminescent QD devices. However, with Samsung’s recent marketing campaign, QLED has now become the de facto term for any display that utilizes QD technology.