These lasers are only as powerful, reliable, and effective as the optics that help to generate, shape, and guide their beams.
High-power lasers have become a standard and ubiquitous tool in many industrial applications, due, in part, to the precisely controllable energy that they provide. But “high power” can be a challenging term to parse, and it often needs some external context to define.
High-power lasers require specially designed mirrors, beam expanders, filters, and lenses that can guide and shape beams without suffering damage in the process. Courtesy of Edmund Optics.
Laser applications are of limited help when qualifying the threshold for high-power output, as laser parameters can range from a 10-W beam in a 3D printer to the 100-kW beams generated by satellite-based lasers. What constitutes an abundance of power for one application would barely register in another. “High power” also becomes a relative term when applied to lasers of different wavelengths, when comparing continuous-wave and pulsed operation, or even when comparing solid-state, gas, or doped-crystal sources.
The same beams that can slice through steel or that can relay telecommunications
signals over immense distances can also wreak havoc on the sensitive optical components that guide and shape light within a laser system’s framework. “You have to control every last little detail, because otherwise that little detail is going to be where the damage starts — and the next thing you know, you’ve blown half a million dollars of components,” said Tim McComb, global business development manager at Coherent.
Quality control
A host of optical elements are required to ensure that a laser’s beam achieves the desired shape, size, and intensity. In addition to lenses for focusing and collimating the beam, a laser system will also often incorporate mirrors, polarizers, and beamsplitters. Each component must be precisely manufactured and machined and then treated with specialized coatings to ensure that the final product has the appropriate light absorbing, transmitting, and reflecting properties. If not carefully monitored, every stage of fabrication, polishing, coating, and testing offers ample opportunities for flaws or errors to slip in that can prompt a system failure.
An engineer holds a beam-shaping module with an integrated microlens system for use in high-power UV laser sources. Courtesy of Focuslight.
One component might have defect sites that basically create a weakness in the overall optical assembly. As a defect absorbs energy that it should be transmitting or reflecting, then the eventual failure of that component propagates through the rest of the system, said Matthew Dabney,
principal laser engineer at Edmund Optics.
High-power laser systems are not cheap. But efforts to cut corners on consumable components will most likely boomerang back on end users, who bear the cost for maintenance, repair, and replacement of the system.
The intense light of a laser can also cause problems via heat-induced deformation of optical components. Even if these effects do not immediately destroy the affected optics, changes can be induced in the refractive index of the material that result in distorted or suboptimal laser output. Laser manufacturers therefore need to be mindful of a range of considerations when specifying optical components for a particular laser system.
First is their choice of material. Fused silica is a very well-characterized glass that exhibits very low absorption and is easy to shape and polish, which often makes it the best choice for many transmissive and reflective optical components.
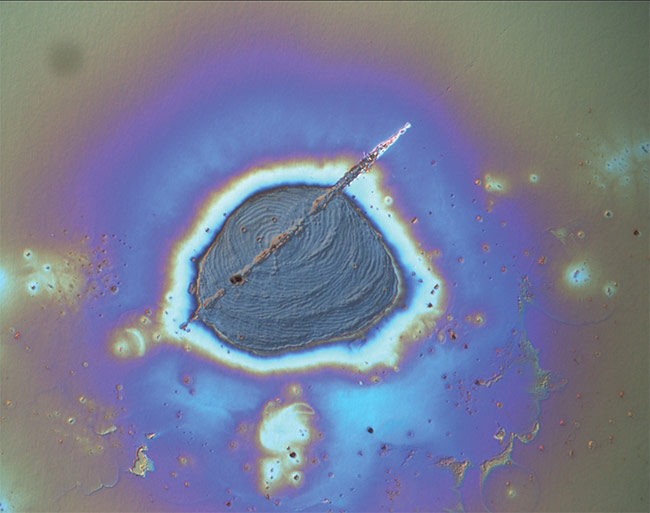
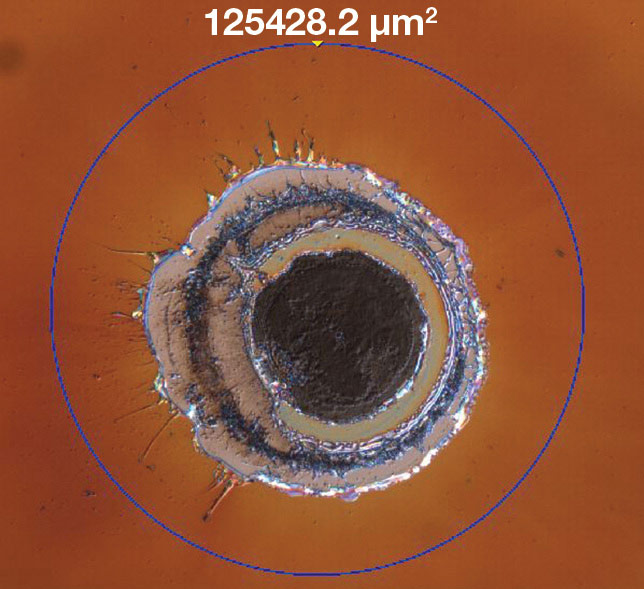
Microscopic images of laser-induced damage on the surfaces of several optical components. Courtesy of Edmund Optics.
“When we go to high-power applications, we always try to use fused silica,” said Dirk Hauschild, R&D director for laser optics at Focuslight. “You can get it with the highest quality levels, and coatings on fused silica have the highest damage thresholds.”
Some laser systems, however, require more specialized alternatives. The lenses in high-power CO2 lasers are often prepared from zinc selenide, which exhibits robust performance under intense infrared light, but it can be more difficult to work with. Like other optical materials, zinc selenide must be precisely shaped and smoothed. Tiny surface defects can prompt performance problems, or worse, due to localized accumulation of energy and heat from the laser. This generates stress to the substrate material that can cause any coatings to break and burn, Hauschild said. “For really high-power applications, a single defect can break the whole optical element.”
Eliminating such flaws entails a meticulous grinding and polishing process followed by careful quality control. Emiliano Ioffe, IR process development and engineering manager at Ophir, said his company typically aims for surface roughness values of <1 nm for its components, with no scratches or digs permitted. This can be particularly challenging when working with nonfused silica materials,
and Ioffe said his team has had to develop specialized polishing processes for the zinc selenide optics employed in the company’s CO2 lasers — particularly when preparing the aspheric optics that have become an increasingly favored lens material.
These flawlessly smooth surfaces must then be uniformly layered with specialized coatings that confer the appropriate reflective or antireflective properties onto the components. Coatings can often be the weakest point of the design, Hauschild said. Because they are very thin, they can break, and they can change material properties over time. As a consequence, a poorly selected or applied coating can undo the hard work invested into producing a perfect lens or mirror.
In addition to their absorbance and reflectance properties, coatings must be selected for optimal performance at a particular wavelength. “For UV, [there are] three or four materials that are typically used, whereas for infrared, there’s a very different set of three or four materials,” Dabney said.
In many cases, components must receive multiple layers of different coatings to improve a desired optical property, but this improvement may come with trade-offs. “You can add more and more layers to raise the reflectance on a mirror, but then as you add your layers, they will also absorb, and so you will lose some of the light to absorption,” Ioffe said. “There is always a balance between absorption and reflectance and transmittance.”
Multilayer coatings must also be carefully designed to avoid the formation of peaks in electric field intensity at the interfaces between layers, which can compromise the integrity of the coating and ultimately cause components to fail.
In search of meaningful metrics
Maintaining high levels of quality control while manufacturing
at commercial scales is no mean feat. For some essential performance metrics, such as absorption, there are no universal standards for companies to work with. “You cannot buy a sample with a specific absorption as a master to calibrate your system,” Ioffe said. “There are systems that we have developed and built in-house in order to measure absorption, phase shift, reflectance, and transmittance in different angles and different polarizations.”
This lack of universal standards is especially problematic for assessing laser-induced damage threshold (LIDT), a metric that describes the maximum level of energy that a given component can be subjected to before it experiences measurable damage.
“There are a couple of ISO [International Organization for Standardization] standards that apply, but those are not sufficient to really create a consistent laser damage threshold test,” said Dabney, who is part of an American National Standards Institute (ANSI) initiative to create more detailed testing standards.
Hauschild further said that these standards may not be well suited for evaluating the LIDT of optics, as new laser designs continue to challenge the performance boundaries for output.
LIDT is directly influenced by the composition, quality, and coating of the optical components themselves, as well as the wavelength and power of the beam they influence. But other factors also come into play. For example, the size and the shape of the beam can shift the threshold based on how much energy is distributed across a given surface area of the component. Some of these factors can be mathematically modeled, but accurate LIDT assessment ultimately requires direct testing of the components themselves.
This is where the lack of LIDT testing standardization becomes problematic. Currently, Dabney said, the ISO standard qualifies LIDT as “noticeable damage.” But this opens the door to disreputable manufacturers marketing optical components with an LIDT that is unrealistically high by keeping their damage assessment procedures at a superficial level. “You’re not rewarded for looking harder — you’re actually penalized,” he said. LIDT should therefore be used as an informative guideline rather than as a target for routine operating conditions. Hauschild said his team typically operates its laser systems at power densities well below the LIDT to ensure long-term stability.
Using power wisely
With great power comes great responsibility, and users must take care to design their laser systems so that a failed component does not create a catastrophe. “One of the big problems is that if people aren’t setting up their beam lines correctly, you can get a feedback loop that takes out the laser,” Dabney said. For example, failure in one component may result in the beam being unexpectedly reflected back to the source by a mirror.
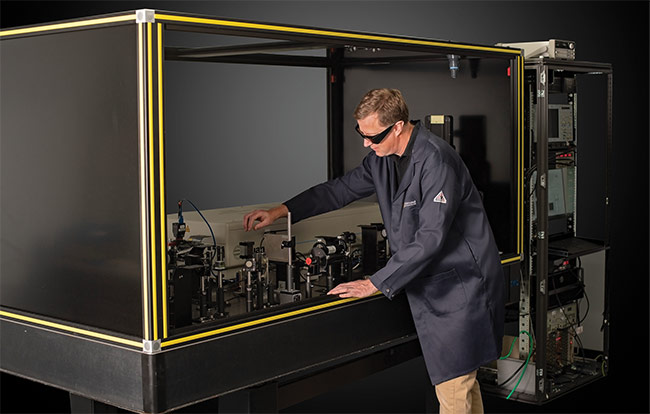
Matthew Dabney, principal laser engineer at Edmund Optics, tests the laser-induced damage threshold of optical components in a specially designed laser lab. Courtesy of Edmund Optics.
“That could take out your $100,000 laser, because of a $100 part,” Dabney said. The laser source should therefore be isolated to prevent such back-reflection events from occurring.
Routine maintenance and monitoring of the laser system and its components is also critical. For example, in materials processing applications, the protective windows on high-power lasers are prone to accumulate contaminants generated by the material being processed, and the windows must be regularly replaced to prevent damage that may enable those same contaminants to infiltrate the laser itself. In addition to running lasers below peak power to minimize stress on the system, Hauschild recommends the use of detectors that can monitor the temperature of the system or that can sense evidence of unexpected light scattering that may forecast an imminent component failure.
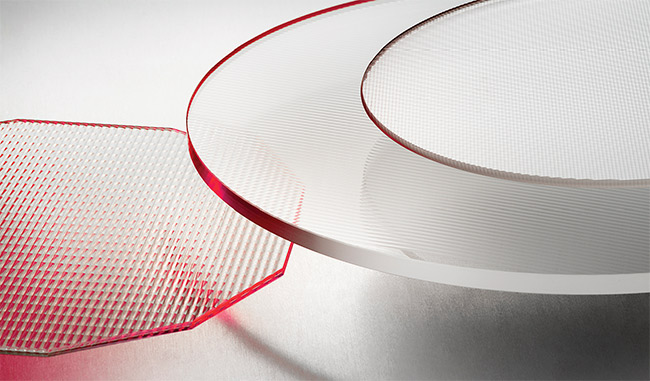
A variety of microlenses produced at wafer scale (top). High-power lasers incorporate combinations of such microlenses with different sizes and focal lengths to achieve the desired beam output. A homogenizer for shaping high-power beams (bottom) Courtesy of Focuslight.
High-power laser systems are not cheap. But efforts to cut corners on consumable components will most likely boomerang back on end users, who bear the cost for maintenance, repair, and replacement of the system.
“If you’re driven by power, then you’re going to pay for it,” Dabney said, but he also noted that this should motivate laser consumers to think twice about how much power they actually need for their particular application. “If you can stay under certain levels, then you can economize a little bit,” he said.
And even though some laser systems will undoubtedly continue to push the power envelope — for example, those designed for defense applications or nuclear fusion research — Hauschild said he also sees the potential for alternative solutions for some industry users. For example, multiple lasers operating at lower peak power may deliver similar productivity at lower cost, in some cases. “The question is not only should we continue to scale power, but how we can use the power in an efficient way,” he said.