The pursuit to harness fusion energy is shifting from fundamental research to engineering. High-power pump laser diodes hold the key as an enabling technology to achieve energy gain.
LUKAS GRUBER, LEONARDO ELECTRONICS US INC.
Fusion energy has promised for decades to offer a clean, carbon-free, safe, and virtually limitless source to power the planet. Now that Lawrence Livermore National Laboratory (LLNL) scientists on Dec. 5, 2022, have achieved “net target gain” at the National Ignition Facility (NIF), this promise is ever more tangible. Net target gain refers to a controlled fusion reaction that produces more energy than was supplied to the target to initiate it. This demonstrated ability to obtain more energy from a controlled fusion reaction than the amount that was supplied — just as it happens in the sun and the stars — reinvigorated the quest for viable fusion reactors.
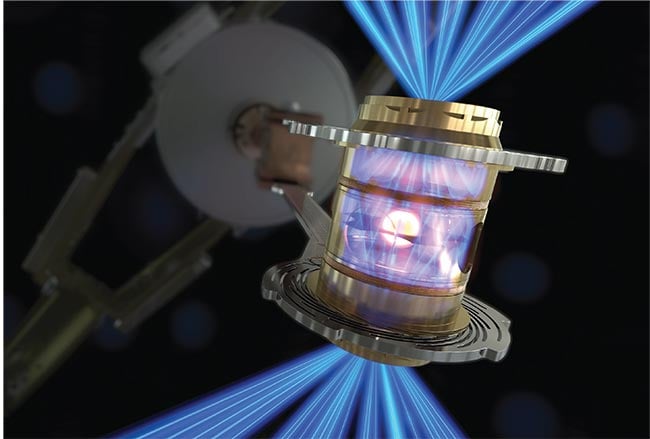
At the National Ignition Facility (NIF), 192 laser beams enter the hohlraum from two sides and strike the inner walls to create x-rays (silver and gold). The massive x-ray wavefront converges
on the deuterium-tritium (D-T) fuel pellet and compresses and heats it (center). Courtesy of Lawrence Livermore National Laboratory (LLNL).
The significance of the NIF team’s achievement cannot be overstated. This advancement not only heightened the interest in the fusion process for the scientific community and public but also demonstrated that the underlying physics of laser-driven inertial fusion energy (LD-IFE) can now be understood. The several subsequent successful ignition shots since December 2022 have reinforced this understanding.
This milestone, as well as many related technical developments that preceded and followed the NIF result, has sparked the private sector’s renewed interest in the fusion space. With the aim of advancing and harnessing the immense commercial potential of fusion energy technologies, numerous companies worldwide are on the path to developing working reactors. Others are targeting the underlying technologies required to enable and improve the next iteration of fusion sources.
In each case, the efforts that are driving fusion science forward are focused on a collaborative, modular approach to achieve new milestones on the road to commercialization. High-power pump diodes are positioned at the heart of these advancements as well as the further development of practical fusion reactors.
Fusion basics
Currently, fusion industry players are
opting to pursue several distinct
approaches to harness fusion. The two main approaches — magnetic confinement fusion (MCF) and inertial confinement fusion (ICF) — are favored by most companies within the fusion space.
The most common mechanism involves fusing nuclei of deuterium (D) and tritium (T) to yield an alpha particle, or helium nucleus, and a free neutron. The total mass of all particles after the reaction decreases slightly compared to the total mass before the reaction. This difference in mass (Δm) is converted directly into energy (E) according to Einstein’s theory of special relativity, E = Δm·c2, where c
is the speed of light. Most of this energy is carried away by the neutron in the form of kinetic energy.
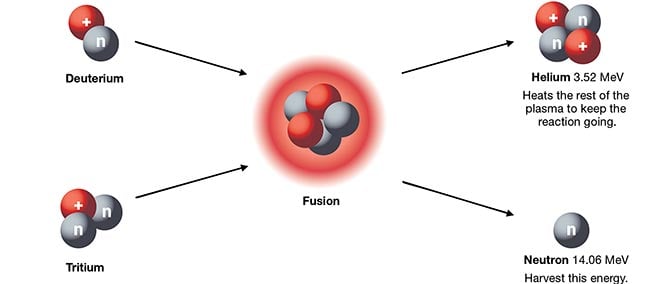
A fusion reaction using deuterium (D) and tritium (T) as fuel, resulting in helium and an energetic neutron as reaction products. The helium nucleus, or alpha particle, typically
contributes to further heating in the fusion plasma and the energetic neutron can be harvested to convert its energy into heat and ultimately drive a steam turbine. Courtesy of Leonardo Electronics US Inc.
Three conditions must be met in a plasma to produce net energy through fusion. First, the positively charged nuclei must be energetic enough to get so close that the strong force overcomes their electrostatic repulsion and draws them together. This requires a sufficiently high temperature. Next, the nuclei must have
a high-enough probability of colliding. This requires a combination of high
density or sufficiently long confinement time.
The considerations around this final condition are multifaceted: If the density
is low, the confinement time must
increase to allow for enough fusion
collisions. If the confinement time is short, then the density must be increased to ensure sufficient interaction between plasma particles. The product of these three parameters — pressure, confinement time, and temperature — called the triple product, must exceed a certain value (the Lawson criterion) to achieve fusion.
The only place in which the Lawson
criterion is met naturally is within stars, where gravitation creates extreme densities and high temperatures, and the confinement time is close to infinity. As a result, building a fusion reactor requires creating conditions that are similar to those occurring at the center of the sun. MCF typically achieves long confinement times but lower densities, whereas ICF tends to have short confinement times but extremely high densities. In both cases, very high temperatures (~10× the temperature of the sun’s core) are required to spur a successful fusion.
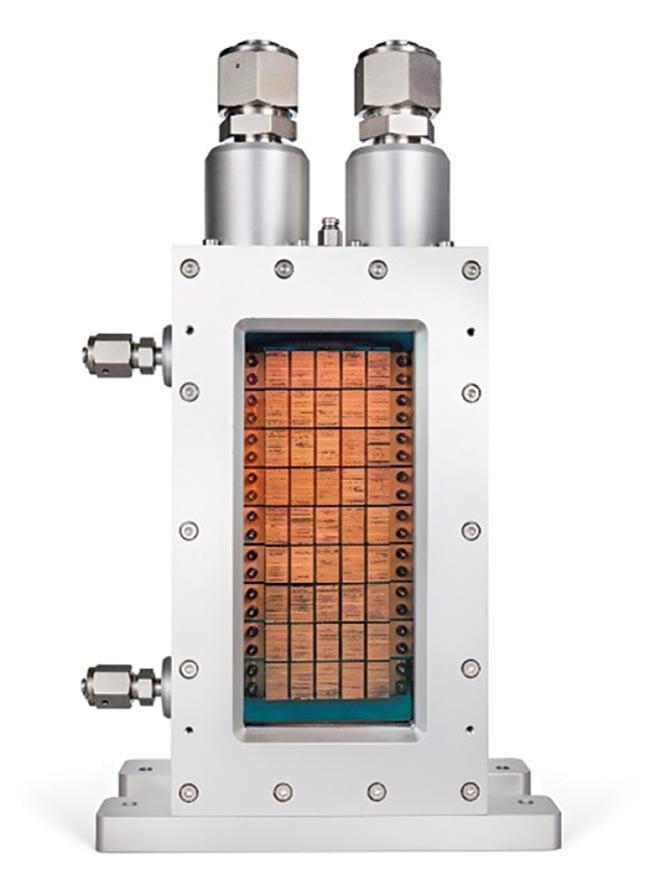
The progression of all ignition shots at the National Ignition Facility (NIF) dating from December 2022 to February 2024. Courtesy of Lawrence Livermore National Laboratory (LLNL).
To meet the Lawson criterion in IFE,
an external energy source, often a very powerful laser, is applied to a small fuel pellet containing the D-T fuel, which becomes rapidly compressed and heated. This causes the pellet to implode and reach enormous pressures and temperatures for brief occurrences, enabling
fusion reactions.
Satisfying the Lawson criterion in
magnetic confinement systems (MFE), such as the tokamak or stellarator, involves using powerful magnetic fields to contain and control a hot plasma. In contrast to LD-IFE, researchers pursuing MFE are in turn pursuing fusion by attaining extended confinement times at low pressure.
For both approaches based on D-T fuel, the alpha particle typically stays in the plasma and contributes its energy, further helping to heat the reaction. The neutron, on the other hand, typically escapes the reaction volume and hits the wall of the chamber. There, its energy can be
harvested and converted to heat.
An industry takes shape
Many technological developments have served to establish market opportunities for companies at all reaches of the commercial fusion landscape. Compact, high-temperature superconducting
magnets, high-peak power laser diodes, and, most critically, net target gain are among the individual technology areas and target goals driving early arrivers to the fusion industry. While their technological approaches and commercial goals vary, each is driven by the pursuit of a share of a multitrillion-dollar energy market.
Simultaneously, dozens of organizations, both public and private, are now actively involved in the development of fusion energy concepts. Participants in these groups include international collaborations and consortia, national laboratories, R&D centers, and private companies.
While the advancements of each industry player contribute to sustained progress in fusion science, individual milestones and benchmarks, in many cases, are longstanding. In MCF, for example, the Joint European Torus (JET) set the record of gain, Q, in a tokamak experiment. The group achieved the high mark of Q = 0.67 in 1997 (compared to Q = 2.34 at the NIF). And today, the JET has been shut down following Brexit as well as to focus on rejuvenation of the U.K.’s fusion program.
More recently, the megaproject of the International Thermonuclear Experimental Reactor (ITER), led by a consortium of 35 countries, is driving steady progress in its build, despite schedule and budget overruns. In industry, the startup company Commonwealth Fusion Systems is a leader in the field, due to funding of more than $2 billion and the successful development of a compact, high-temperature superconducting magnet that holds promise for achieving energy gain in a much smaller device footprint.
On the ICF side, beyond the 2022 achievement of ignition and net target gain by the NIF, four subsequent repetitions of this achievement since the flagship result have sustained the momentum. This wave of excitement and success is reaching the industry sector, where California-based Longview Fusion
Systems is applying the same physics used for the NIF result. The company aims to improve the efficiency of the laser driver in its technical approach by replacing flash lamps with laser diodes. Additional startups targeting ICF and using efficient high-peak power laser diodes include Focused Energy, Marvel Fusion, and HB11 Energy.
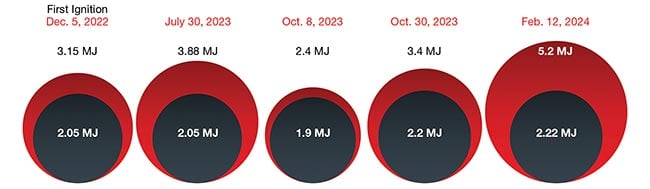
A 900-kW laser diode module built in 2016 by Leonardo, as it is used to pump the main amplifier in the High-Repetition-Rate Advanced Petawatt Laser System (HAPLS) installed at Extreme Light Infrastructure (ELI) Beamlines in Prague. Courtesy of Leonardo Electronics US Inc.
Amid the launch and the recent
success of members of the fusion industry, the Fusion Industry Association (FIA) is facilitating the process of enabling commercial fusion energy use to become a reality. The FIA, encouraged by the
plurality of approaches, is aiming to bridge fusion companies, organizations in the fusion supply chain, investors, regulators, and policymakers. The FIA brings fusion companies under its umbrella as well as the supply chain feeding this industry.
Consortia: the road map to practical fusion
As the FIA provides an organized
approach to the complex regulatory
and policy backbone of this nascent industry, the technological developments themselves require an organized path toward successful commercialization of product technologies and solutions.
Although fusion efforts of various reach are now active in Australia, Canada, China, France, Germany, Italy, Japan, Russia, South Korea, the U.K., and the U.S., the collaborative nature of the industry suggests that leadership in one facet
of fusion science is apt to benefit the entirety of the industry.
The 2022 White House summit on “Developing a Bold Decadal Vision for Commercial Fusion Energy,” for example, preceded the announcement by the U.S. Department of Energy (DOE) of its plans to fund three hubs to accelerate IFE
science and technology. These hubs comprise members from academic institutions, national and international labs, commercial entities, philanthropic organizations, and private IFE companies. The hubs are the Science & Technology Accelerated Research for Fusion Innovation & Reactor Engineering (STARFIRE), led by LLNL; the Inertial Fusion Energy-Consortium on Laser-Plasma Interaction (LPI) Research (IFE-COLoR), led by the University of Rochester’s Laboratory for Laser Energetics (LLE); and the Inertial Fusion Science and Technology (RISE), led by Colorado State University. While each hub focuses principally on many
different aspects of fusion, the use of
resources provided by the different
entities is a common thread. This multi-pronged approach demonstrates the importance of pursuing fusion with the expertise and resources from diverse stakeholders.
Similarly, this past spring, Japan announced the creation of a consortium as part of its Moonshot program. The initiative will include government organizations and private companies with the shared goal of developing advanced technologies in fusion and defining a path for commercialization. In April, Germany unveiled the PriFUSIO consortium, led by the Fraunhofer Institute for Laser Technology ILT (Fraunhofer ILT). The effort unites fusion startups, medium- and large-size companies, and semipublic
research centers to advance their missions. Critically, not all members are solely pursuing advancements in fusion science — a fact that highlights the importance and shared pursuit of fusion science progress across a variety of disciplines.
While the funding for these consortia is modest, their operation represents a multifaceted approach, providing crucial
expertise in many technical aspects. These consortia can serve as nucleation points for idea-sharing and discussion on how to best approach the commercialization of IFE. Additional collaborations can also emerge from these groups, which may provide this blossoming industry
with added direction, especially in identifying cost drivers, pursuing the standardization of technical solutions, and advocating for relevant investments.
Next steps
The seminal achievement of target gain in laser-driven IFE answered difficult physics questions for this specific approach and has signaled a degree of optimism within the fusion community. Still, several technological questions are unanswered in the domain of LD-IFE, and, indeed, for all fusion approaches.
To advance LD-IFE specifically,
companies and organizations must improve the cost and manufacturability of targets; develop appropriate target injection and tracking methodologies; mitigate optics damage; and improve laser diode cost.
Still, several prominent technology questions must be answered for all the
approaches. Experts must find, for
example, the optimal materials for the
reactor wall (first wall) that faces the fusion reaction and is most heavily bombarded with neutrons. They will also need to validate solutions for the fuel cycle at reactor scale. As a result, educating a workforce to sustain this industry is a pressing matter.
With the relevant consortia in place, legitimate opportunity exists to facilitate and create industry standards to govern the use of technologies that are required in fusion power plants. Such standards can align a variety of individual solutions and create efficiencies in supply chain, manufacturing technologies, and devices. This consolidation of solutions should subsequently drive cost savings through economy of scale.
Ultimately, to move the industry forward in the most substantial fashion, a facility is needed to serve as a proving ground to explore existing technologies,
develop new ways to solve existing
questions, and exercise the supply chain. A recent call for papers from the DOE’s Office of Fusion Energy Science triggered a multitude of white papers focused on this need. The final selection for funding did not include any laser-driven facilities, though new funding opportunities are continuing to be announced, and the industry is simultaneously gaining momentum.
The importance of diodes
One key limitation in the NIF laser system, and other extreme-power laser sources, directly involves the technology used for pumping gain media. The amplifier stages of the NIF laser are pumped with flashlamps — a relatively inefficient method, and one that produces large amounts of waste heat.
Replacing flashlamps in a NIF-like facility with laser diode pumping is an achievable step toward raising the
efficiency of the entire system by at least one order of magnitude to a level that may enable energy break-even. The increased efficiency and thus reduced waste heat allows the operation of the laser system at the required shot rate of 10 Hz or more, compared to 1 Hz, or much less, for
flashlamp pumping. It should also be noted that laser diodes are expected to represent the single most significant cost of an item in such a facility. As a result, the future of LD-IFE (incorporating diode-pumped, solid-state lasers) is dominated by both the cost and performance success of laser diodes.
Fortunately, progress in this technology
area is underway. For example, in 2016, Leonardo delivered five laser diode
modules, each with 800-kW output, to the High-Repetition-Rate Advanced Petawatt Laser System (HAPLS) project. This laser is designed to produce petawatt pulses with an energy of 30 J or more and pulse widths of <30 fs at a 10-Hz repetition rate. At the time of this delivery, the technology and performance of the HAPLS laser diode arrays marked a significant technological leap. Progress has only
continued since then. If Leonardo were
to build HAPLS-like laser diode arrays
today, these arrays would be able to deliver >2 MW each with an unchanged form factor.
Fusion research has not been the only driver behind the development of advanced laser diode sources such as these. As with many other areas of laser and photonics technology, the defense industry, as well as science applications, created a pull that equally moved the technology forward, benefiting the fusion industry.
Fusion in reach
The pursuit of fusion energy, once a scientific curiosity, is now a burgeoning industry that is on the brink of revolutionizing global energy. With groundbreaking achievements, such as the net target gain at the NIF, paired with advancements in laser diode technology, the pathway to practical fusion energy is clearer than ever. Collaborative efforts from international consortia, private sector investments, and technological innovations are accelerating progress.
As a result, the fusion industry holds the promise of providing safe, abundant, and carbon-free energy. Industry players and those offering their solutions and support continue to tackle the relevant technological challenges. The future of fusion energy shines brightly, heralding an era of sustainable power.
Meet the author
Lukas Gruber is a director of business
development at Leonardo Electronics US Inc. With more than 25 years of experience in the photonics industry, he has held many positions, ranging from fundamental research at Lawrence Livermore National Laboratory to product development, manufacturing, and general management; email: [email protected].