New materials, improved tooling and innovative design bring plastic optics to the forefront of defense, biometrics applications and more.
Paul Tolley, Syntec Technologies Inc.
For many years, polymer optics languished as the stepchild of the photonics world. Considered unsuitable for high-precision applications, plastic lenses were relegated to toys, low-accuracy eyepieces and low-end cameras — markets where high volumes, intense cost pressures and space constraints made these materials the only viable solution. With continued improvement in both materials and manufacturing techniques, new markets emerged, first in telecommunications and then in biomedicine and biometrics. These industries demanded more of optics than low cost. Also critical were high precision, complete reliability, exceptional versatility and single-use flexibility.
To meet these needs, suppliers developed new materials, insert manufacturing techniques and ways to produce smaller and smaller tooling. Most importantly, they worked with designers to integrate multiple optical and mechanical elements into repeatable components and assemblies. As a result, plastics are also taking center stage in homeland security and military operations.
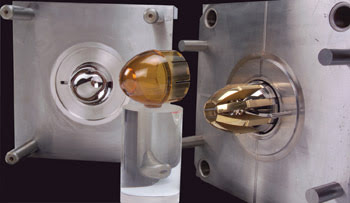
Manufacturing techniques, such as injection molding, allow optimized volume production of plastic optics for diverse applications from toys to these bomb guidance domes.
As early as World War II, the US made serious attempts to use plastic optics in military applications, but efforts were limited by available materials, primitive tooling capabilities and process quality. In the 1960s, plastic optics for cameras broke the mold to become cost-competitive with glass optics. Low-cost fixed-focus cameras required repeatable lenses of significant quality at volumes and price points impossible to achieve with glass. By the mid-1990s, plastic optics systems had found their way into such diverse technologies as fiber optics, surgical laparoscopic devices, image scanners and laser-guided bombs.
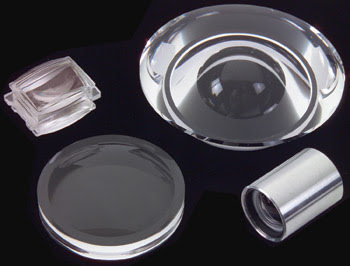
Fueled by continuing advances in materials and processing technology, plastic optics offer relatively low weight and cost, combined with good optical characteristics, which promotes their use in military applications such as laser-guided bombs missile guidance optics.
Today’s high-growth opportunities include the following:
• Biomedicine, including blood assay diagnostics and disposable surgical and treatment devices.
• Biometrics, including three-dimensional facial imaging, readers of palm- and fingerprints and retinal scanners.
• Defense and homeland security, including guided weapons, head-up displays and identification and tracking devices.
Engineering advances
Plastic optics continue to prove their worth in the precision-guided weapons and other military systems. Raytheon’s Paveway laser-guided bomb, for instance, uses plastic lenses to acquire targets because of their relatively low weight and a cost five times lower than their predecessors. Use of plastic optics in head-mounted displays reduces the weight of the imaging system by 8 ounces, minimizing user fatigue. Moreover, in the broadband systems for defense and industrial communications alike, mass-producible polymer connectors transfer information as reliably as glass, again at a fraction of the cost.
When space constraints and optical complexity converge, plastic optics may be the only option. Consider biometric devices, such as facial and fingerprint identification systems, where plastic optics solutions combine design versatility with high repeatability and low cost.
In one such system, the object plane had to be compacted to fit a 3.5-mm height constraint. As a result, the unit needed a main element with three diffractive surfaces: an off-axis parabola coated for second-surface reflectivity, an aspheric cylinder and two ellipses for illumination dispersion. It also required a biaspheric focus lens and a first-surface folding mirror. Meeting these space constraints would have been impossible, except with plastic optics.
Similarly, satisfying one-time-use requirements cost-effectively virtually requires plastic optics. For example, the advent of blood-borne pathogen diseases drove the initial need for blood assay analyzers with a disposable platform. Typically, illumination and imaging elements must be combined in one disposable package. Because total-internal-reflectance principles apply readily to polymer designs, it is possible to design relatively low-cost multisurface elements that satisfy both excitation and imaging requirements. In addition, all the common optical plastics, including acrylic, polystyrene and polycarbonate, have high transmission in the visible to the near-IR wavelengths, which meets the needs of such devices.
As a point of interest, recent initiatives to detect Severe Acute Respiratory Syndrome — commonly known as SARS — were largely performed with Bayer’s Rapid Point blood gas analyzers, which use plastic lenses for their optic trains and test substrates.
Production technology trends
Modern production technology capabilities for polymer optics are characterized by several factors. Besides new material options, such as cyclic olefin polymers and copolymers, these include advances in the surface generation complexity of optics inserts, few if any boundaries in designing multielement components, and growing capabilities for optics molding by traditional plastics manufacturers.
In most cases, cost still drives the exploration into plastics. Although initial tooling expenses can cause sticker shock, they can be managed. Costs will vary depending on finished component size, number of cavities, number and complexity of optical surfaces, and plastic delivery method (cold runner vs. hot runner).
More importantly, tooling expenses are usually offset, frequently dramatically, by other considerations. First, raw material is cheaper; resin availability lends itself to production needs, especially in high volume. Second, optical design freedom makes it possible to use aspheric or diffractive surfaces or to combine multiple optical surfaces into one element. This translates into reductions in the number of components as well as overall product size. Third, there is unique potential for integrating mechanical design components with optical ones.
The largest area for potential cost savings comes from design for manufacturability, so it is critical to involve the manufacturer early in both overall and specific design objectives. For example, by building mechanical housing or mounting features directly into optical elements, companies save on two levels: manufacturing and assembly. Eliminating spacers and/or extra mounting components simplifies parts handling, reducing scrap and overall assembly cycle time, and streamlines molding.
Optics materials trends
Although advances in polymer materials stagnated for many years, several recent breakthroughs have greatly expanded earlier options for temperature ranges, transmission quality and stability of the index of refraction. As a result, there are many more ways to address particular application needs.
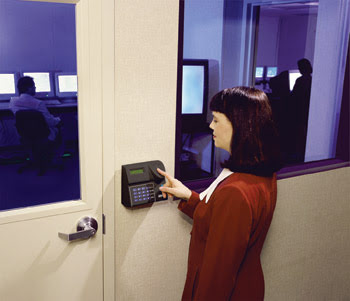
Nonmilitary applications for polymer materials include blood assay diagnostic devices and biometric palm- and fingerprint identification systems.
Polyetheramides and polyethersulfones are now available in optical grades. These plastics exhibit higher transmission quality than previously possible in the near-IR and withstand operating temperatures from below zero to above 210 °C. Several grades of acrylic also deliver relatively high transmission quality from 300 to 1600 nm with improved temperature operating ranges to minimize thermal expansion. Finally, current cyclic olefin products feature extremely low moisture absorption. The issues of moisture absorption and thermal expansion relate directly to the stability of the index of refraction shift with respect to temperature.
Obviously, materials should be selected for baseline optical performance relative to the design, but each polymer class offers numerous grades that address characteristics of flow, purity and environmental durability. Specific information is readily available from the resin manufacturers and molders.
Birefringence, where light separates into two diverging beams as it passes through a doubly refracting optic, is not related to any specific material but remains an important material consideration. It can have disastrous effects on polymer systems that are sensitive to polarization. Because birefringence effects are process-driven, materials with higher flow rates are less susceptible.
Component design is also a factor. The harder a component is to fill (which requires more injection pressure) or pack (more holding pressure), the more likely the occurrence of birefringence. Several postmolding operations, however, can be implemented to reduce its effects.
Tooling and processing
Advances in mold tooling have been most significant in the insert manufacturing process. Using high-quality nickel-plating on hardened steel inserts, single-point diamond turning technology can machine a substrate to a finished surface with an accuracy of a micro-inch or less. Surface roughness is less than 20 Å on nonferrous metals and less than 80 Å on directly turned polymers — significantly higher accuracy than with traditional grind-and-polish methods.
Single-point diamond turning also lends itself to the applications of aspheres, diffractives and nonrotational symmetric optics. This is important to note because the narrow range of index and dispersion values that once made plastic systems unsuitable can be rectified with the use of diffractives. The diffractive grating can reduce the number of elements or spacing required in a system as well as provide critical color correction.
Tooling has also progressed with the use of more advanced unit systems that use a common base. Customers pay only for cavity blocks and inserts. The obviously lower cost of the mold is not the primary advantage of these systems. Rather, it is the shorter lead times that standardization makes possible. A more intricate full-frame mold may require around eight weeks to complete, but a typical unit system can be delivered in as little as three to five weeks.
Polymer processing has also realized extraordinary gains in the past decade. Injection-molding machines are available with closed-loop high-speed microprocessors and multistage injection systems. This enables the process engineer to wield far greater control over the key attributes of the process, including injection speed and injection pressure of the polymer flow as well holding pressures. With the digital technology available today, the technician has significant insight into transition points in the molding sequence. Understanding and monitoring these points eliminates variation in surface geometry from part to part.
As with glass optics, thin-film coatings can enhance image quality. They are applied using the same methods, generally to adjust reflective properties of surfaces. In some cases, additional hardness or durability coatings will increase abrasion resistance.
Plastic optics still need to improve overall in relation to thermal stability, transmission at wavelengths below 300 nm and above 2 μm, and the degree of abrasion resistance. Although there is a flurry of research activity to overcome these hurdles, it’s hard to defy physics.
The greatest limitation to plastic systems remains the index of refraction shift with respect to temperature (dn/dT). In some applications, this can create undesirable aberration and/or shift in focal length. As previously stated, this is due in part to moisture absorption, low glass transition temperature and thermal expansion rates that are generally higher than glass. While several polymers can operate in the 200 °C range, overall environmental conditions must be carefully considered during system design. Spectral range is also limited for polymers. Transmission beyond 1800 nm is erratic and typically unacceptable for high-accuracy systems that work in the mid- and long-wave IR.
So why should optics designers look more intensely at plastics? First and foremost, with the continual merging of global markets and economies, plastic optics will increasingly offer a cost that is hard to beat. Second, many applications previously better served by glass are now suitable for plastics. Developing advances in materials, tooling and processing continue to reduce the effects of thermal changes and distortion, allowing tighter tolerancing and broader operational spectral ranges. Third, growing expertise in designing for manufacturability is leading to ever more optics applications that, while impossible with glass, are not only possible, but also cost-effective for plastics.
As the new respect for polymer optics takes hold, there is little doubt that these systems will contribute not just to demanding homeland security and defense applications, but also to a host of everyday needs.
Meet the author
Paul Tolley is vice president and general manager of Syntec Technologies Inc. in Pavilion, N.Y. Active in SPIE, he is a member of its Technical Advisory Committee for Homeland Security.