With continued fine-tuning, optogenetics may lead to better treatments of once-implacable maladies that affect
muscle coordination, sleep and vision.
Controlling a biological response with a shock of light is a somewhat recent development.
Most people flinch when intense light is flashed across their eyes. Over the eons, this strong reaction has provoked scientific curiosity, and that curiosity has brought about a deep knowledge of how the retina responds to light and ignites a sequence that ends with an image sent to the brain. But only during the current century has genetic manipulation resulted in the production of highly specific light-reactive proteins that drive muscle movement, neuronal activity or other desirable actions.
Light-based techniques are not unfamiliar to Samarendra K. Mohanty, an assistant professor at the University of Texas at Arlington who frequently has used optical tweezers and other laser-based tools to manipulate neurons. The comparatively low power requirements of optogenetic techniques attracted him to attempt modulation of neurons in vivo, starting with a new approach to retinitis pigmentosa (RP).
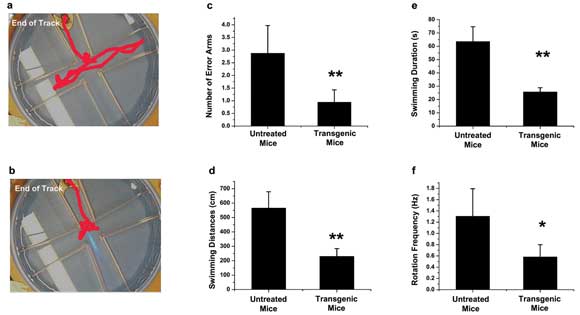
In a model of retinitis pigmentosa, mice transfected with channelrhodopsin-2 and exposed to an optimally designed dose of blue light perform better at a sight-dependent water maze challenge. (a) The representative path that a nontreated mouse took to solve the maze; (b) the representative path taken by a transfected mouse; (c) the number of error arms mice swam inside on their way to the destination platform;
(d) the swimming distance before reaching the destination platform; (e) the swimming duration before successfully finding the platform; and (f) the frequency with which the mice rotated their heads while seeking their destination. Courtesy of Samarendra Mohanty, University of Texas.
RP is a degenerative condition that affects the sight of about 1 million people worldwide. Individuals with the condition typically experience night blindness as the rods that comprise part of the retina begin to deteriorate from the outside in. In many sufferers, the retina’s cones also become affected, which presents as tunnel vision that narrows as time passes. In all, vision slowly becomes dimmer over many years, and color sensitivity is lost completely.
The current standard treatment for RP is artificial retinal implants or natural transplantations. However, researchers believe that rods and cones can be regenerated, or other cells nearby enhanced to act as rods and cones, through optogenetics.
Higher-order neurons such as retinal ganglia can be made light-sensitive, Mohanty said. The altered cells can be stimulated with patterned light to form images inside the visual cortex. Today’s state-of-the-art approach uses channelrhodopsin-2 as the photoactive molecule to engage retinal neurons in their new tasks. Channelrhodopsin-2 works under blue light (about 450 to 480 nm), which results in monochromatic sight. Mohanty discovered that these opsins can respond to infrared light but require light too high in intensity to be practical.
In mice, Mohanty’s team transfected cells inside the eye with channelrhodopsin-2 (tagged with yellow fluorescent protein for easier viewing of protein expression).
The traditional way to deliver opsin-generating genes is to use virus-mediated transfection, in which a microneedle injects a viral load near the target site. Mohanty’s group used electroporation to deliver the opsin genes to retinal ganglion cells. A different technique, developed by the group, uses a laser-driven analog of electroporation to open more gentle pathways into the cells in desired areas of the retina.
“Unlike our laser transfection method,” Mohanty said, “viral transfection of [the] retina will perturb the active neuronal circuitry in the central part of the retina.”
One week after transfection, the investigators tested the ability of the mouse subjects to traverse a radial-arm water maze. The mice were rewarded if they successfully responded to a light-based stimulus while crossing the maze.
In testing the efficiency of channelrhodopsin-2 expression, the researchers alternated light sources, using a 473-nm diode-pumped solid-state laser made by LaserMate Group Inc. of Pomona, Calif.; an LED array from Thorlabs Inc. of Newton, N.J.; and microLEDs from Rohm Semiconductor USA LLC of San Diego. (The team recently developed microLED arrays and a fiber-array-based prosthetic that can generate stimulation patterns for the retina or, in the case of an enucleated eye or a damaged optic nerve, the visual cortex.)
The investigators found that the mice transfected with channelrhodopsin-2 performed much better than those that did not receive the treatment, successfully navigating the maze by a 3:1 ratio.
Whereas channelrhodopsin-2 responds solely to blue wavelengths, the researchers are looking to expand their repertoire into new opsins that are sensitive to green and red. But the main challenge, Mohanty said, is in obtaining the opsins that have the proper temporal kinetics to restore vision in real time.
“In [the] future, with synthesis of new opsins sensitive to green and red (and near-IR) wavelengths, and microLED-array-based prosthetics, we hope that true (high-resolution and color) vision can be restored,” Mohanty said.
The rhodopsins, including channelrhodopsin-2, are G protein-coupled receptors (GPCRs), well-known modulators of neurons that often are considered in the search for new pharmaceuticals for a variety of neurological and cardiac disorders. Besides their effect on the visual system, GPCRs also often have a quelling effect on other electrophysical reactions, such as motor coordination.
GPCRs are found throughout the brain and are expected to play a large role in brain-directed motor control. This control is suspected to be acted out via large Purkinje cells, through which the cerebellar cortex sends messages to the body’s musculature. One’s balance and coordination, for example, depend on the rhythm and pattern of electrical firings of the Purkinje cells, and GPCRs help play the tune to which the cells dance.
Improving one’s grip
Testing how GPCRs affect Purkinje cells typically is done through pharmacology, using chemicals to change the pattern and cadence. Unfortunately, this tends to affect GPCRs in other cells in the area as well, possibly skewing results. Researchers at Ruhr University in Bochum, Germany, led by Stefan Herlitze, were dissatisfied with the inherent issues that come with pharmacological methods, so they turned to an optogenetic approach.
Herlitze and his colleagues created a mouse model that expressed vertebrate rhodopsin (vRh), a light-sensitive protein that can be expressed in specific cells, including Purkinje cells. Using a laser from CrystaLaser of Reno, Nev., the investigators fired 26-s pulses through a fiber optic mounted onto the head of each mouse, delivering 473-nm light to the cerebellar region. Control mice had vRh but received no laser light stimulation. The mice were then tested in feats of grip strength, balance and motor coordination.
Switching gears
Mice that expressed vRh after each new pulse showed clearly altered motor abilities. In the future, however, the group would like to further investigate whether individual motor functions have separate signaling pathways that could be addressed independently through photoactivation of vRh.
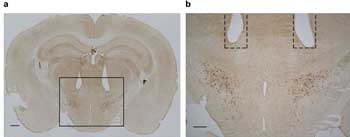
In Akihiro Yamanaka’s test of halorhodopsin expression in orexin neurons, the researchers confirmed the location of the fiber optic tips within the mouse brain. The orexin neurons are shown in brown within the lateral hypothalamic area of the brain. (a) Scale = 500 µm; (b) scale = 300 µm. Courtesy of the Journal of Neuroscience.
Orexin, known also as hypocretin, is a neuropeptide involved with several brain-directed activities, including food cravings, sleep regulation and wakefulness. It also appears to have a role in the sleep-related pathology narcolepsy. Increases in orexin are known to increase wakefulness and decrease rapid eye movement and “slow-wave sleep,” colloquially known as deep sleep.
Akihiro Yamanaka and his colleagues at the Japanese National Institute for Physiological Sciences in Okazaki wanted to determine the mechanism of action behind orexin’s effect on wakefulness.
So they created transgenic mice in which orexin neurons expressed photo-reactive halorhodopsin, which responds to orange light. They used a xenon lamp with a 586 ±10-nm filter made by Semrock Inc. of Rochester, N.Y. They controlled light intensity with neutral-density filters, delivering the light via fiber optics directly connected to the mouse subjects’ brains.
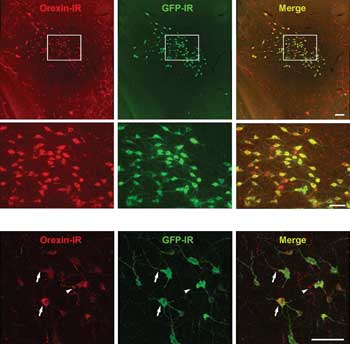
Halorhodopsin is expressed in orexin neurons in these examples of transgenic mouse brain. The halorhodopsin was not expressed elsewhere, whereas the GFP was expressed also in the soma cells and dendrites. Scale = 100 µm. Courtesy of the Journal of Neuroscience.
Shining orange light on the cells with halorhodopsin inhibited orexin neuron discharge, with the amount of inhibition tied to the intensity of the excitation light. This silencing, when invoked in the transgenic mice during daylight hours – when the mice were naturally inactive – caused changes in brain activity characteristic of slow-wave sleep.
In contrast, the excitation light, at any intensity, had an effect during nighttime hours, when the mice were active. Ultimately, the team was able to control sleep induction by using halorhodopsin’s ability to silence orexin under the orange light.
Yamanaka and his colleagues believe that further investigation into the role of orexin neurons may reveal more about the mechanisms of symptom progression in narcolepsy patients.