Scientists call for deeper imaging at a higher frame rate with a wider field of view, but can the top microscope makers rise to the challenge?
Of the numerous far-field imaging techniques, multiphoton microscopy has proved particularly valuable in many biological studies, providing multimodal images from deep within tissue, as well as label-free images of complex biological structures and inorganic probes.
From fluorescence microscopy for imaging living animals and label-free techniques such as second-harmonic generation and coherent anti-Stokes Raman scattering spectroscopy for imaging tumors in intact tissues together with the increasing use of next-generation femtosecond laser platforms to further advance neuro-imaging and in vivo brain studies, there are numerous multiphoton methods that have been developed since the concept was demonstrated over two decades ago.

This is an egg-stage 22 Gryllus bimaculatus (cricket) embryo, stained with phalloidin coupled to Alexa488. The image was acquired using multiphoton excitation at 900 nm and LSM 880 with Airyscan in Fast mode as the detection device (www.extavourlab.com). Courtesy of Cassandra Extavour and Seth Donoughe, Harvard University, USA.
Common to all of these methods is the mechanism in which two or more photons combine to generate a contrast difference within the sample resulting in optically sectioned images with high resolution. Another commonality is that multiphoton microscopy users would like to see some developments that could improve the quality and impact of their research and even help bring the technique into the clinical setting.
At King’s College London, professor Klaus Suhling and colleague Simon Ameer-Beg are finding innovative ways to speed up multiphoton imaging for beam-scanning applications. In a paper published in Biomedical Optics Express (https://doi.org/10.1364/BOE.6.000277), the team, led by Ameer-Beg, demonstrated that multifocal multiphoton imaging with a 2D single-photon avalanche diode array offers significant speed advantages compared with single-beam laser scanning or wide-field fluorescence lifetime imaging (FLIM) microscopes.
“We have recently used multibeam-scanning multiphoton microscopy for rapid acquisition of FLIM data based on time-correlated single-photon counting,” Suhling said. “The use of multiple beams and multiple detectors has allowed us to speed up data acquisition significantly, by more than an order of magnitude, whilst retaining single photon sensitivity.”
But to routinely make FLIM movies and study dynamic processes quantitatively, Suhling says the team would need compact femtosecond laser sources, tunable over a large wavelength range, as well as better detectors.
Echoing the call for smaller sources is professor Stanley Botchway at the Science and Technology Facilities Council and Oxford Brookes University in Oxford, England. Through his research, he also highlights that the need for a multidisciplinary approach is now more important than ever.
Within the Science and Technology Facilities Council, Botchway and his team are using multiphoton microscopes to investigate drugs such as combretastatins used for age-related macular degeneration, phototherapy and brain cells imaging using multiphoton processes. Their research is now at the stage of deep tissue imaging of live mice1.
For real-time disease detection and treatment, starting with animal models, Botchway says that very long working distance objectives — beyond 10 cm — would be needed as well as multiple outputs from the laser platform. This would enable selective multicomponent imaging with significant excitation of the different fluorescent probes under investigation.
“Multiphoton microscopy hasn’t changed much in the last 10 years. But lasers for multiphoton microscopy have improved significantly with ease of use and tunability,” he said. “Although small footprint femtosecond lasers would allow them to enter clinical settings.”
Microscope manufacturers are keen to respond. Some of the leading microscopy specialists are already working to overcome the top challenges set forth by modern day science.
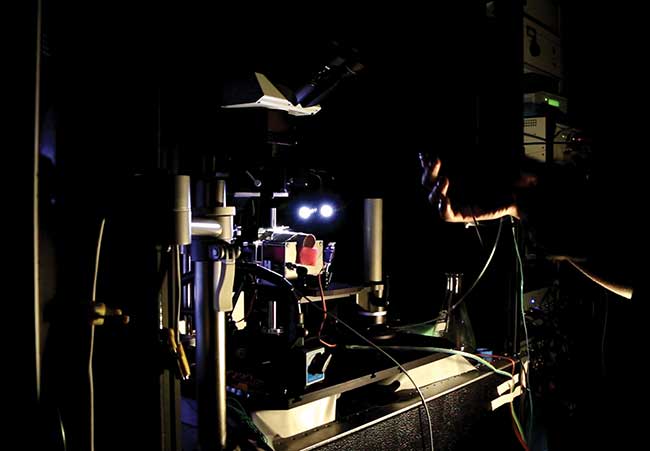
Researchers prepare a multiphoton microscope for in vivo imaging in mice. Courtesy of Scientifica Ltd.
Increasing imaging depth
Increasing the penetration depth in vivo remains one of the primary challenges for neuroscientists. Researchers hope to study neuronal activity deep in the cortex and the hippocampus of mice, in some cases do away with implanted cranial windows in favor of imaging through the intact skull, and image the brain of larger rodents and possibly small primates.
The difficulty is combining the use of lasers emitting at longer wavelengths, where water absorption does not affect the sample, delivering larger amounts of energy per pulse and the use of adaptive optics for removing the detrimental effects caused by aberrations due to the sample structure.

In vivo 6DPF zebrafish larvae expressing GCamp6 imaged using the Scientifica HyperScope. Courtesy of Scientifica Ltd.
In this case, concepts applied in astronomy to measure atmospheric turbulence have been brought into microscopy successfully2 to measure and correct for the aberrations inside the sample by using the nonlinear guide star concept. Third-harmonic generation has also been used to perform long-term time-lapse recordings of more than five hours in living samples3.
Some of today’s leading biophotonics and neuro-imaging laboratories are turning to three-photon excited fluorescence (3PEF) microscopy and third-harmonic generation microscopy, using longer IR excited wavelengths, typically 1.3 µm for green markers and 1.7 µm for IR markers.
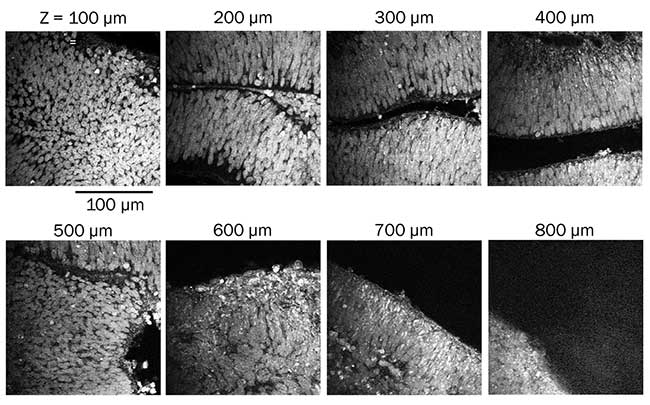
Combined three-photon excited fluorescence and third-harmonic generation imaging of DAPI-stained mutant cerebral organoid, imaged with Spirit 1040-16 and Spirit-NOPA-IR. Courtesy of Murat Yildirim and Mriganka Sur Laboratory, Picower Institute for Learning and Memory, MIT.
“These techniques typically require high peak powers (greater than megawatt level), moderate average powers (tens or hundreds of milliwatts) at megahertz repetition rate,” explained Julien Klein, senior manager of Product Marketing at Spectra-Physics Inc., in Santa Clara, Calif. “The first practical use of 3PEF for in vivo calcium imaging in live mice was recently demonstrated using a Spectra-Physics ytterbium-based femtosecond amplifier and a noncollinear optical parametric amplifier with high peak power output in the infrared tunable from 1.2 to 2.5 µm.”
At Uckfield, England-based multiphoton imaging specialist Scientifica Ltd., Christian Wilms, an applications scientist, points out that of all the long-term goals, deeper imaging remains the most elusive since the depth obtainable with two-photon imaging is limited by a number of factors.
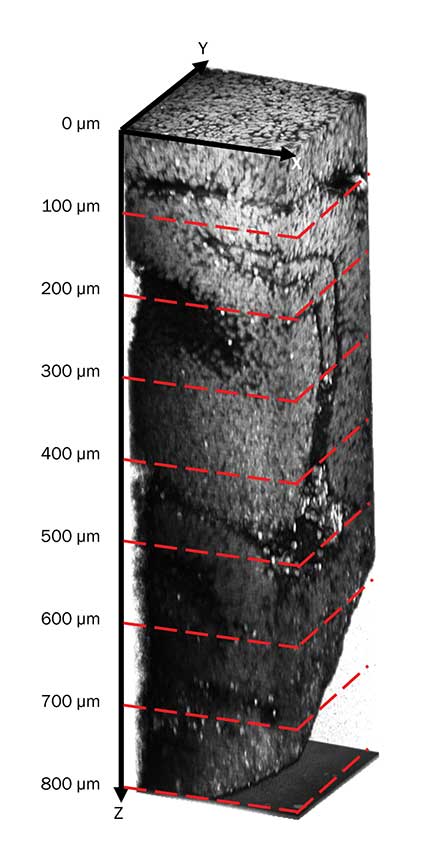
Courtesy of Murat Yildirim and Mriganka Sur Laboratory, Picower Institute for Learning and Memory, MIT.
“Scattering and absorption of excitation light are an unchangeable constraint. In addition, aberrations due to structures in the tissue and refractive index mismatch lead to a degradation of the focal volume2,” he said. “Over the past 10 years several biophotonics labs have tried applying different adaptive optics strategies to this problem. So far there does not appear to be a consensus approach and we anticipate further developments in the future.”
Faster frame rate
Increasing the frame rate to allow researchers to follow real-time processes such as the propagation of electrical activity within the brain is now being
addressed by commercial instruments. For example, the wide commercial availability of resonance-scanning-based multiphoton microscopes has made high frame rate imaging available to all researchers in the past four to five years.
What is more, there is growing interest in acousto-optic deflector (AOD)-based microscopes, to allow for even higher data acquisition rates.
“These advances have been made possible by adding electro-optical devices that allow light to be deflected using a radio frequency signal and a medium that responds to such emission by experiencing a change in refractive index or by using techniques that allow whole volume imaging such as wavefront coding4,” explained Rodrigo Aviles, Electro-Mechanical development manager at Scientifica Ltd.
Wider field of view
Increasing the field of view size while maintaining subcellular resolution is particularly important for neuroscientists, enabling them to investigate how different regions of the brain communicate with each other. First commercial systems allowing fields of view up to 5 mm were introduced in November 2016.
Compact femtosecond laser sources
According to Eva Simbürger, product manager of Light Microscopy at Carl Zeiss Microscopy GmbH based in Jena, Germany, there has been good progress in providing compact femtosecond laser sources thanks to the development of laser technologies already used in telecommunications.
“Compact fiber lasers will be a (near) future option if not already used,” she said. One drawback, however, is that they are not tunable. But on the other hand they have a very small footprint and already provide reasonable output power.
The use of alternative ultrafast laser technologies has already been demonstrated in the past, such as a semiconductor disk laser emitting at 965 nm used for simultaneous two-photon excited fluorescence and second-harmonic generation in both fixed and living samples5 and chip-sized devices based on quantum dot technology emitting around 1260 nm used to demonstrate its use for two-photon excited fluorescence imaging6.
Multiple tunable sources for multimodality
The use of more laser lines in parallel is becoming more and more prominent. As researchers look into the use of even higher wavelengths, manufacturers are triggered into developing optics that provide a reasonable transmission — particularly in the range of 1100 to 1500 nm or above.
“With the extended tuning range of ultrafast lasers and microscopes that are now able to efficiently transmit further IR-shifted excitation light, this is definitely an avenue that we expect to grow quickly in the coming years,” Scientifica’s Wilms said.

A dual scan-head multiphoton microscope. Courtesy of Scientifica Ltd.
For example, a 30-fs laser system tunable around 1 micron has been demonstrated for use on living samples7. The system uses a microstructure fiber to broaden the spectrum of a Yb:tungstate, solid-state laser.
In fact, today’s multimodal microscopes now contain input ports for ultrashort pulsed lasers to allow for the combination of multiple techniques such as two-photon-excited fluorescence, second-harmonic generation, third-harmonic generation and coherent anti-Stokes Raman scattering.
Higher resolution
According to Carl Zeiss Microscopy’s Simbürger, there are ideas to achieve higher resolution and sensitivity for intravital imaging, and the combination of multiphoton imaging with new Airyscan technology has provided a rather good starting point to achieve this.
“Intravital imaging on awake animals using optogenetic approaches now will trigger much more imaging with direct feedback on behavior,” she said. “This will definitely expand, although those experiments are very demanding.”
Taking a different approach is materials scientist and professor Jeff Squier, who heads up the Department of Physics at the Colorado School of Mines. Squier is working with professors Randy Bartels and Jeff Field from Colorado State University to develop a new enhanced resolution imaging method that works across multiple imaging modalities and requires only single-element detection.
Their work was reported in PNAS (PNAS, doi: 10.1073/pnas.1602811113) in May 2016 and details a method based on spatial-frequency-modulated imaging for multiphoton superresolved imaging that allows for simultaneous imaging of both coherent and incoherent signal light.
“We have developed a general method for enhanced resolution that is applicable across multiple imaging modalities, second-harmonic generation imaging, two-photon excitation fluorescence, etc.,” explained Squier. “The really exciting concept is that it is easy to deploy — existing multiphoton microscopes can be readily adapted to use this method.”
References
1. K.M. Scherer, et al. (May 13, 2016). New approaches to photodynamic therapy from type I, II and III to type IV using one or more photons. Anticancer Agents Med Chem.
2. R. Aviles-Espinosa, et al. (2011). Measurement and correction of in vivo sample aberrations employing a nonlinear guide-star in two-photon excited fluorescence microscopy. Biomed Opt Express, Vol. 2, Issue 11, p. 3135, doi.org/10.1364/BOE.2.003135.
3. R. Aviles-Espinosa, et al. (2010). Third-harmonic generation for the study of Caenorhabditis elegans embryogenesis. J Biomed Opt, Vol 15, Issue 4, doi:10.1117/1.3477535.
4. O.E Olarte, et al. (2015). Decoupled illumination detection in light sheet microscopy for fast volumetric imaging. Optica, Vol. 2, Issue 8, p. 702, doi.org/10.1364/OPTICA.2.000702.
5. R. Aviles-Espinosa, et al. (2011). Compact ultrafast semiconductor disk laser: Targeting GFP based nonlinear applications in living organisms Biomed Opt Express, Vol. 2, Issue 4, p. 739, doi.org/10.1364/BOE.2.000739.
6. Y. Ding, et al. (2012). High peak-power picosecond pulse generation at 1.26 µm using a quantum-dot-based external-cavity mode-locked laser and tapered optical amplifier. Opt Express, Vol. 20, Issue 13, p. 14308, doi.org/10.1364/OE.20.014308.
7. B. Resan, et al. (2014). Two-photon fluorescence imaging with 30 fs laser system tunable around 1 micron. Opt Express, Vol. 22, Issue 13, p. 16456, doi.org/10.1364/OE.22.016456.
Multiphoton Microscope: A User’s Wish List
• Increase the imaging depth. Requires the use of lasers emitting in the absorption window for excitation of biological tissue. In this region, water absorption does not affect the sample and optical scattering is lower.
• Increase the frame rate. To allow for the following of real-time processes.

Operational schematic of the multifocal multiphoton system. Courtesy of Dr. Simon Ameer-Beg, King’s College London.
• Extend the field of view employing customized optical paths and large-scale optics. To allow wider fields to be visualized to a few millimeters.
• Develop compact femtosecond laser sources. Mass clinical uptake is hampered by bulky femtosecond lasers operating between 680 to 930 nm. The clinical setting would need small footprint, simple turnkey femtosecond lasers at this wavelength and below (600 to 640 nm) for the excitation of UV-absorbing photodynamic drugs.
• Include multiple tunable sources for multimodality. Single box tunability from 500 to 1300 nm would be excellent as currently an optical parametric oscillator (and ring cavity) is needed to cover below 680 nm.
• Increase resolution. To enhance the quality of research as well as open the door to new imaging applications.