In a year that has seen the biggest recession in decades hit most market segments, you might expect a look back at the optics industry in 2009 to be all gloom and doom. But there are some bright spots in the darkness, where cutting-edge research and emerging applications are forging a promising future in many optics sectors.
For instance, research into novel non-linear optical effects for image contrast in biology and medicine is one of today’s hottest topics in optics. As professor Chris B. Schaffer of Cornell University in Ithaca, N.Y., explains, “Nonlinear optical imaging has become the technique of choice for in vivo microscopy studies in biomedical research. The combination of high-contrast, molecularly specific [with appropriate labels] and high-resolution imaging deep into tissue is unmatched by other microscopy methods.”
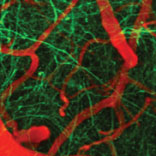
In this two-photon-excited fluorescence image of neurons (green) and blood vessels (red) in the brain of an anesthetized mouse, the blood vessels are labeled with an intravenous injection of fluorescent dye. The images were acquired on a custom-built two-photon microscope in Chris B. Schaffer’s laboratory, using a fiber laser constructed in Frank W. Wise’s laboratory that produces 100-fs pulses at 1030 nm. Courtesy of Matt Farrar and Chris B. Schaffer, Cornell University.
One of the most successful imaging methods is two-photon-excited fluorescence microscopy, a technique first demonstrated in professor Watt W. Webb’s laboratory at Cornell in 1990 that allows micron-resolution imaging of living tissue up to a depth of 1 mm.
In the approach, a structure of interest is labeled within a tissue using a fluorescent dye. Next, high-intensity pulses from an infrared femtosecond laser are tightly focused into the sample, causing localized excitation of the fluorescent molecule. Two photons from the laser pulse can interact with a single fluorescent molecule simultaneously, leading to the excitation of light emission and of an electron, but only in the focal volume where the intensity is sufficiently high. The emitted fluorescence can be detected and an image formed by scanning the position of the laser focus through the sample in three dimensions, a technique often called laser-scanning microscopy.
The crucial element that sets it apart from other fluorescence-based imaging techniques is the highly localized excitation. “Fluorescence is excited only in the [micron-size] focal volume and nowhere else in the sample,” Schaffer explained. “Because of this, you can be sure that any fluorescence light you detect must have come from the focal volume, no matter how much that fluorescence scatters in the tissue on its way to the detector.”
Looking beyond imaging
Most exciting about nonlinear optics are the many avenues of research being explored in laboratories worldwide. Thanks to this research, there were several notable advances in the field in 2009.
One of the most important takes advantage of new laser sources such as fiber, opening the field to clinical applications. Fiber lasers offer crucial advantages over their solid-state counterparts, including compact size and robust performance, which are useful for biological imaging and ocular surgery. Recently, researchers such as professor Frank W. Wise at Cornell have achieved a level of performance from fiber-based femtosecond lasers that meets or exceeds that of their much more cumbersome and expensive counterparts.
A significant advance in nonlinear microscopy took place in October 2009. Professor X. Sunney Xie at Harvard University in Cambridge, Mass., published work detailing the development of stimulated emission microscopy, opening the door to nonlinear spectroscopy applications in biology. The technique allows label-free imaging of molecules that absorb but do not fluoresce. The sensitivity of the technique is orders of magnitude higher than that for spontaneous emission or absorption contrast and could lead to possibilities for biomedical imaging, including label-free mapping of drug distribution and blood vessels in tissue.
At another US research camp last year, professor Mark J. Schnitzer and colleagues at Stanford University in California invented two forms of fiber optic fluorescence imaging, dubbed one- and two-photon fluorescence microendoscopy. By creating portable, miniaturized microendoscopy devices based on flexible fiber optics, the team can carry out minimally invasive in vivo imaging of cells in deep brain areas of freely moving mice. This remarkable feat could shed light on how cellular properties are affected by environment, training or life experience.
With so much varied research, the possibilities seem endless. According to Schaffer, there will be an increasing trend toward longer-wavelength nonlinear microscopy, which enables deeper imaging. He also predicts an explosion of new nonlinear contrast mechanisms that will allow important quantitative information to be gathered.
A silent underground revolution is under way. Beneath our feet lie hundreds of tiny bundles of fiber, streaming information, images, videos and much more directly into our homes. As consumer demand moves from simply surfing Web pages and downloading a few songs, to watching high-definition television (HDTV) and uploading home movies into e-mails or Web pages, the need for greater bandwidth bears down on service providers.
Fiber optics: The race is on
Sam Bucci, vice president of optical networks at Alcatel-Lucent in Ottawa, said that these companies are struggling to squeeze ever-higher bandwidth out of their technologies. “In 2009, bandwidth demand continued to grow at a significant rate, but service providers’ profitability has not grown at the same rate. Network upgrades must be met with transport solutions that significantly lower total cost of ownership. This includes capital and ongoing operational expenses such as power, which must be achieved without sacrificing quality of service or reliability.”
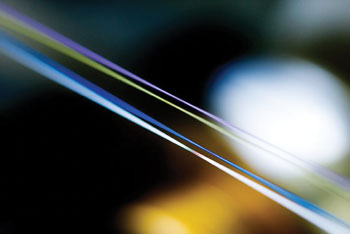
Shown is an example of Alcatel’s optical fiber after the coloring process. Courtesy of Alcatel-Lucent.
For an idea of today’s network demands, a standard-definition television signal requires a bandwidth of about 2 Mb per second. HDTV requires as little as 4 Mb per second if the image is rather static; for example, as a person is being interviewed. But fast action, such as sporting events, requires more – as much as 8 Mb per second, even with new compression technology such as MPEG4.
Already being used in some academic and industrial settings is 3-D immersive HDTV, which is expected to hit the consumer market next year with the launch of Panasonic Corp.’s Full HD 3D Home Theater system. Such high-demand technology will require between 100 and 300 Mb per second. Current fiber optics technology can provide two-way transmission speeds of up to 100 Mb per second.
Easing traffic congestion
Just how will service providers cope with increasing demand on their networks? An emerging trend in the industry is the concept of Internet Protocol (IP) offloading. Just as vehicles can travel long distances more quickly via an interstate highway system, so, too, can light be transmitted more directly using the idea of offloading. “Traffic in the core of the network is mostly transitory and, as such, could be more economically handled at lower layers instead of bogging down valuable router resources,” Bucci said. “The concept involves processing transitory traffic at the Optical Transport Network or [at the] DWDM [dense wavelength division multiplexing] layers, which can provide significant power savings.”
In and out of Africa
In July 2009, a $600 million project involving 17,000 km of undersea fiber optic cable was completed, providing countries in East Africa with access to international broadband networks for the first time.
Brian Herlihy, CEO of Seacom, the company responsible for the project, said that Africa now can launch itself as a major competitor for call center/business process outsourcing. “Furthermore, clinical research that must share real-time data with hospitals around the world will now be possible, allowing science, education and other research to be carried out from anywhere in eastern or southern Africa as easily as it could be conducted in Cambridge, Massachusetts.”
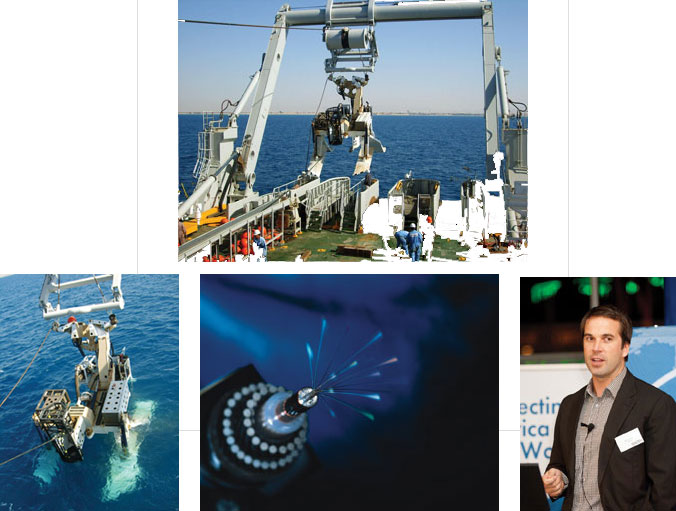
Images at top and far left show Seacom’s burial plow being hoisted and then lowered into the sea. The huge machinery prepares the way for the company’s optical fiber cables. Left center, Seacom’s project involved the installation of 17,000 km of undersea fiber optic cable, providing countries in East Africa with access to international broadband networks for the first time. Brian Herlihy, at left, has extensive experience in mega-infrastructure projects in Africa spanning disciplines including project development, financing and governmental liaison. Images courtesy of Seacom.
Bringing high-demand services in and out of Africa is Seacom’s cable, which is composed of two fiber pairs with a total capacity of 1.28 Tb per second. For the first time, consumers in East and South Africa can enjoy high-speed Internet, peer-to-peer networks such as Skype, HDTV and IP television.
“We have given African communications carriers equal and open access to inexpensive bandwidth, removing the international infrastructure bottleneck and supporting east and southern African economic growth,” Herlihy said. “In Kenya, we have already witnessed the birth of three … national networks, South Africa is developing four national networks, and Rwanda is rolling out huge fiber networks throughout the country.”
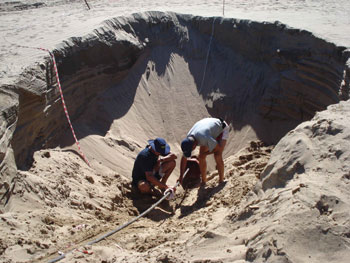
Seacom’s pull-through bore pipe helps engineers bury the cable underground. Courtesy of Seacom.
High-brightness LEDs display their true colors
High-brightness LEDs have been one of the most dynamic sectors of optoelectronics over the past 10 years. Dr. Robert V. Steele, director of optoelectronics at Strategies Unlimited in Mountain View, Calif., said the market grew from $800 million to $5.1 billion between 1999 and 2008, driven primarily by adoption in automotive, mobile electronics, outdoor signage and, more recently, lighting applications.
Applications for high-brightness LEDs are many and varied. Because they are as much as 100 times brighter than conventional LEDs, they are most suited for outdoor use. They can be found in traffic signals, pedestrian signals/controls, vehicle headlights and advertising billboards.
Although conventional LEDs can be used for indoor displays, their color range is very limited. “There are no blue conventional LEDs, and there is no true green – the green ones are actually greenish-yellow,” Steele said. “So if full color displays or video screens are needed, requiring red, green and blue LEDs, then high-brightness LEDs must be used.”
According to a study carried out by ElectroniCast Consultants, a market forecasting firm in Upper Lake, Calif., high-brightness and ultrahigh-brightness LEDs held 69 percent of the worldwide market share in terms of US dollar value in 2008 versus 31 percent attributed to conventional LEDs, which are used in solid-state general lighting applications. The report predicts that, by 2015, high-brightness and ultrahigh-brightness LEDs will hold a 97 percent global market share in solid-state general lighting applications.
Stephen Montgomery, president of international business expansion at ElectroniCast, said the main drivers of this remarkable growth will come from maintenance/labor, safety/security and “green tech” issues.
This year has seen a major uptake of high-brightness LEDs in backlights for LCD displays in notebook computers, televisions, monitors and in general lighting. In fact, the entire high-brightness LED market is growing so fast that Steele predicts it will reach $14.9 billion in 2013.
For LED manufacturers and lighting specialists such as Osram Opto Semiconductors GmbH of Regensburg, Germany, the decline in the automotive and consumer electronics sectors has had a direct effect on business. But it is not all bad news, thanks to new markets opening in which high-brightness LEDs are prized for their size, lifetime performance, full-color quality and high-quality white light.
“[High-brightness] LEDs are already well established in some market segments such as architectural lighting, decoration/beautification and signage,” said Dr. Michael Fiebig, Osram’s marketing and business development manager for LEDs and organic LEDs. “In other segments, the breakthrough is about to take place step-by-step, notably in outdoor lighting, professional shop lighting and residential lighting.”
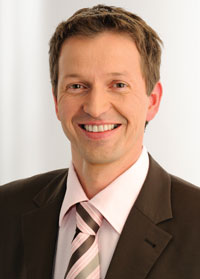
Dr. Michael Fiebig heads up marketing and business development of LEDs and organic LEDs in the solid-state lighting segment at Osram Opto Semiconductors GmbH. Courtesy of Osram Opto Semiconductors GmbH.
The first projects are already under way in street and tunnel lighting and in lighting for city squares. Although the cost of high-brightness LEDs compared with conventional lamp technologies remains elusively high, Fiebig expects LED solutions to conquer mass markets within the next three years.
A clear picture of adaptive optics
Adaptive optics can be traced back to 1953, when the idea of improving the performance of optical systems by reducing the effects of optical distortion was first proposed by US astronomer Horace W. Babcock. But it was not until four decades later, in the 1990s, that adaptive optics became widely used when advances in computer technology made the technique practical.
Today, adaptive optics can be seen at work both on the largest and smallest scales, including astronomy, medicine (such as retinal imaging) and for military purposes (such as rangefinding). More recently, adaptive optics has been used to enhance the performance of free-spaceoptical communications systems, including the project under way at the US Air Force Research Laboratory in Rome, N.Y.
The challenge with free-space optical links between humans on and near the surface of Earth is the turbulence-induced distortions on information-bearing electromagnetic waves. Temperature gradients cause wind, and this wind is not always smoothly varying. In a sense, it boils. This effect can be seen in the form of mirages when we view distant objects across a hot plain, or ahead of us on a scorching highway. Using adaptive optics to eliminate distortion will provide the military with ultrahigh-bandwidth intelligence, surveillance and reconnaissance information in real time.
So far, Air Force researchers have established an optical link at distances exceeding 9 km in both stationary and flight situations. The next step is to test the system at higher altitudes to demonstrate greater air-to-ground distances.
At the opposite end of the spectrum, adaptive optics is making waves in microscopy. For example, remarkable work on male fertility as well as work producing 3-D snapshot images to track particles in live-cell biology are under way at Heriot-Watt University in Edinburgh, UK. Professor Alan H. Greenaway’s team exploits two adaptive optic wavefront sensing technologies – phase diversity and image sharpness – in the same measurement to deliver depth measurements approaching l/100-in. wide-field microscopy. The researchers currently obtain a depth resolution better than 10 nm, but with further improvements in photon-counting detector technology, they believe that angstrom-level resolution – required in molecular studies – is possible.
The group’s 4-D sperm imaging project, which started in November 2009, examines the complex tail movement of sperm cells. Funded by the UK’s Science and Technology Facilities Council, the project aims to contribute to important studies on human fertility.
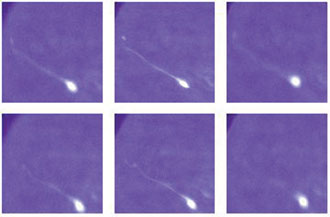
Shown are two consecutive sets of in-focus images of sperm swimming. Preliminary data was taken under negative phase-contrast illumination in collaboration with Dr. Jackson Kirkman-Brown and Dr. Dave Smith, both of the University of Birmingham in the UK, and Jez Graham of Cairn Research Ltd. Depth separation between in-focus planes – i.e., between images along rows – is 910 nm. Note the propagation of a knotlike structure along the tail and toward the head. Courtesy of Heriot-Watt University in collaboration with the University of Birmingham.
For a clear idea on where adaptive optics technology is headed, it seems we will have to be patient. Until more efficient modulators and wavefront sensors are developed, Greenaway expects a pause in the evolution of adaptive optics systems.
“I believe that a point will come where a combination of these technologies in a complete adaptive optics system will suddenly open new possibilities and challenges, but the impetus to improve these components is first needed,” he said.