Remus T Dame, Leiden University, Leiden, and Vu University, Amsterdam, the Netherlands, and Joost Van Mameren, JPK Instruments AG, Berlin
Over the past few years, optical tweezers increasingly have been used to answer questions of biological significance. Doing a search with key words such as “optical tweezers” and “optical trap” on the Web of Science database shows that, over the past decade, the number of articles containing these terms has increased exponentially.
However, another clear trend can be observed: No longer is the mere application of optical tweezers sufficient to have an article published in a high-impact journal. It is increasingly important to generate knowledge that is useful and relevant to understanding biology. This automatically begs for a shift in players. For many years, the field has been crowded with physicists and engineers who were driving technological developments while investigating biological systems alongside. Now the time seems ripe for a deeper involvement of biologists and biochemists.
Molecular motors and DNA
The International Symposium on Optical Tweezers in Life Sciences, which took place in May in Berlin, brought together life scientists covering various applications of optical tweezers (Figure 1). Most speakers were trained originally in physics, reflecting the currently limited diffusion of these techniques among biologists and biochemists.
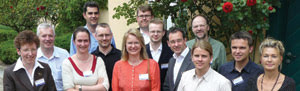
Figure 1. Pictured are speakers at and organizers of the first meeting of the International Symposium on Optical Tweezers in Life Sciences. From left to right: A. Robitzki, J. Molloy, M. Dogterom, M. Hegner, T. Jähnke, L. Oddershede, G. Behme, H. Eggert, S.-P. Heyn, E.-L. Florin, E. Schäffer, A. Rohrbach and C. Friedrichsen.
Microtubules are stiff dynamic protein polymers, the ends of which can grow or shrink. Marileen Dogterom’s group at the Institute for Atomic and Molecular Physics in Amsterdam, the Netherlands, is investigating this process by attaching microtubules to beads that can be trapped with optical tweezers. These microtubules are then aimed toward a microfabricated wall, and the optical trap measures the displacement of the bead upon microtubule growth or shrinkage. Purified dynein protein immobilized on the surface of the microfabricated walls was shown to exert a pulling force on the end of the microtubule.
At the Medical Research Council in London, Justin Molloy’s group is exploring the movement of motor proteins along actin filaments. The experiments carried out in his lab consist of an actin filament suspended between two beads held in optical traps. By using these traps, the actin filament can be exactly positioned on top of a third surface-attached bead coated with myosin motors (Figure 2), which consist of two “legs” and “feet” that interact with actin. The researchers detected the activity, step size and other characteristics of the myosin motors as the movement of the actin filament displaced the trapped beads.
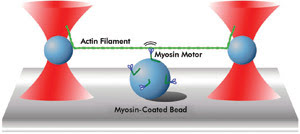
Figure 2. This diagram represents a motor protein motility assay as performed by Justin Molloy’s group at the Medical Research Council in London. A single actin filament is suspended between two optically trapped beads and brought into the proximity of a surface-immobilized bead coated with myosin motor proteins. Motor pulling activity is transduced to the trapped beads, which can be accurately measured.
Martin Hegner of Trinity College in Dublin, Ireland, discussed his recent work on a protein that is exported by a bacterium and infects tobacco plants. He attached beads to the ends of a single-stranded DNA molecule. One of these beads was trapped using optical tweezers, while the second was captured with the suction from a micropipette. Using a clamp to exert a constant force on the protein-DNA complex, he demonstrated that protein binds strongly to single-stranded DNA, which may be essential to promote uptake of the DNA into the host cell.
Whereas the experiments discussed above evolved around purified components in well-defined in vitro systems, the research presented by Lene Oddershede at Niels Bohr Institute in Copenhagen, Denmark, and Alexander Rohrbach at the University of Freiburg in Germany, focused on processes occurring in the context of live cells.
Oddershede reported on the diffusion of a single protein, the so-called lambda receptor, in the membrane of an Escherichia coli bacterium by its attachment to a polystyrene bead. Unlike most of the other work reported at the meeting, the study relied on a very weak optical trap to follow the diffusional motion of the protein and attached bead within the membrane, rather than on a stiff optical trap to capture the bead. From the motion recorded, values for the diffusion constant could be determined. This data led to the notable conclusion that the diffusivity of membrane proteins is dependent upon whether the cell is alive or dead, indicating that diffusion is an active process in the membranes of live cells.
Rohrbach discussed his experiments on phagocytosis, a process in which bacteria or particles are consumed by white blood cells as part of the immune response. Instead of real bacteria, the investigators employed polystyrene beads that were held in an optical trap and brought in contact with “filopodia” tentacles extending from the white blood cell (Figure 3). Individual tentacles retracted in discrete steps, pulling the bead along, and finally acted as a lever arm, delivering the particle to the macrophage surface.
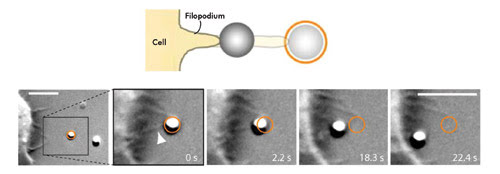
Figure 3. Shown is a model of phagocytosis as studied using optical tweezers. An optically trapped bead attaches to a macrophage filopodium, which subsequently reels in the particle. Figure adapted from Kress et al., PNAS, 2007. © (2007) National Academy of Sciences, USA.
In general, it is difficult to perform experiments in vitro with protein complexes consisting of many individual components, but this is now feasible even with optical tweezers. The observation of active transcription by eukaryotic RNA polymerase was one such major achievement. Possibly even more of a challenge is reconstituting the activity of ribosomes, the large complexes responsible for translation of mRNA into proteins. This challenge was recently met: Researchers were able to see the progression of the ribosome along the mRNA, one building block at a time.
Advances reported
While these studies addressed primarily the properties of the proteins binding to DNA or RNA, several other recent studies have reported large technical advances in new directions. RNA molecules are known to fold back onto themselves to yield a secondary, highly complex structure, which recently has been seen to undergo complex folding and unfolding dynamics by exerting a well-controlled force on the RNA molecule. In a recent study of Rad51, a protein involved in the repair of damaged DNA in humans, investigators employed optical tweezers to manipulate the DNA molecule while directly visualizing the DNA-bound Rad51 enzymes labeled with a fluorescent dye. The authors of this work thus managed to observe the disassembly of Rad51-DNA filaments and to follow the dissociation of individual Rad51 proteins. A final technical “tour de force” was the construction of an optical tweezers instrument with four optical traps that allowed for the simultaneous manipulation of two single DNA molecules. This technique facilitated studies of a bacterial chromatin protein capable of making bridges between two DNA molecules and revealed the size, strength and ordering of adjacent H-NS bridges.
A first commercial platform
At the meeting in Berlin, JPK Instruments presented the NanoTracker, one of the first commercial optical tweezer platforms (Figure 4). The device makes possible the entire range of experiments discussed by the other speakers at the symposium.
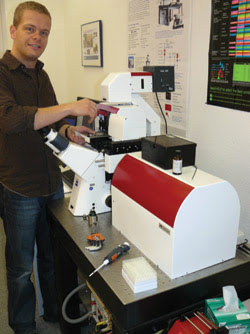
Figure 4. Co-author Joost van Mameren operates JPK’s NanoTracker, one of the first commercially available optical tweezer platforms.
The technical advances in optical tweezers, promoted over the past decade by academics with strong physics backgrounds, have brought the technique to a state in which it may become a laboratory tool rather than one for research and development alone. Reliable, accurate and stable measurements are now performed routinely by increasing numbers of life sciences researchers worldwide. The way has been paved for the development of commercial optical tweezer platforms, which are expected to spread use of the technique rapidly. The concurrent trend of moving from method-driven to question-driven research is thus likely to be further emphasized over the coming years.
Meet the authors
Remus T. Dame is an assistant professor at Leiden University in Leiden and at VU University in Amsterdam, and Joost van Mameren is an applications scientist at JPK Instruments AG in Berlin.
References for this article are available upon request.