OCT systems can differentiate between skin lesions and other dermatological conditions, and new approaches seek to enhance existing instrumentation.
Adam Wax and David Miller, Lumedica
The skin is the largest organ of the body, serving as a protective layer against environmental factors that include infection, ultraviolet radiation, and temperature fluctuations. The skin is composed of three main layers — the epidermis, dermis, and hypodermis — and the thickness and composition of these layers varies from 0.3 to 2.6 mm throughout the body. Tissue biopsy is the diagnostic standard of care for many types of dermatological conditions. This procedure involves excising a tissue sample and sending it to a pathology lab where it is sectioned, stained, and imaged under a microscope. This process is invasive to the patient, often resulting in scarring and a risk of infection. Furthermore, this process introduces a delay in treatment — a delay that technologies, such as optical coherence tomography (OCT), can potentially shorten significantly.
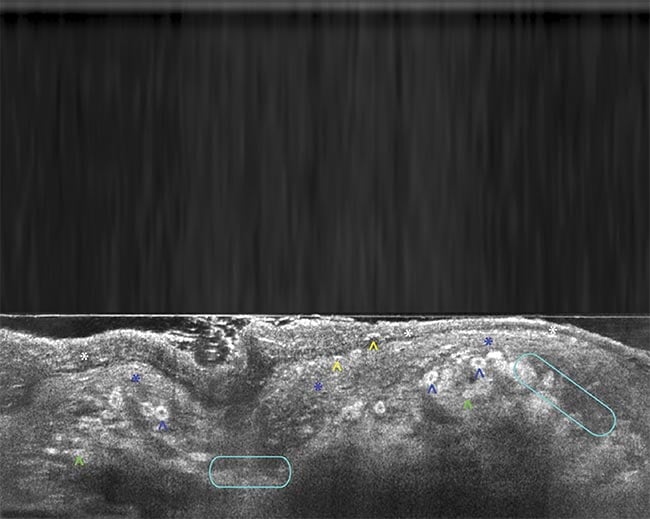
A line-field confocal optical coherence tomography (LC-OCT) B-scan image of melanoma. Adapted with permission from Reference 3.
Indeed, OCT is a compelling imaging modality for dermatology and biomedicine due to its capability to noninvasively create 3D cross-sectional images of biological tissues with micrometer-scale resolution. OCT imaging can penetrate up to 1-mm deep in tissues across a lateral range of ~1 cm. Today’s OCT systems provide >100-dB dynamic range and acquisition speeds that permit real-time imaging. State-of-the-art research systems can achieve 1- to 10-μm axial resolution with most commercial implementations producing 7- to 15-μm resolution. While OCT is extensively used in ophthalmology for retinal imaging, with a variety of commercial devices available, its application in other medical fields, including gastroenterology, cardiology, and dermatology, is still developing; only a few systems are currently approved for clinical use.
OCT for tissue analysis
The operating principle of OCT can be drawn in parallel with that of ultrasound imaging, although the effects being analyzed are different. Light waves are sent into biological tissue and the time delay of the echo can be analyzed to reconstruct a depth-resolved OCT image. However, the speed of light is significantly faster than that of sound, which necessitates the use of interferometry techniques to accurately quantify the time delay.
OCT uses a broadband, low-coherence light source that allows for precise depth selection with high axial resolution within tissue, such as the skin. This interferometric approach can achieve a dynamic range >100 dB, allowing for penetration depths of 1 mm or more, depending on the tissue properties and wavelength. Additionally, the choice of wavelength and detection approach, along with advanced beam geometries and analysis techniques, can further improve diagnostic capabilities for assessing tissue structures at various depths.
The microscopic imaging capabilities of OCT have led to its investigation as an optical biopsy technique, a method for visualizing the microscopic structure of tissue without requiring tissue excision, processing, or staining. OCT saw rapid adoption in ophthalmology for identifying and characterizing the structure of the retina, where biopsy is nearly impossible without disrupting its function. It is this capability that aligns OCT with use in dermatology.
Despite this, the adoption of OCT within dermatology has been slow. This is largely due to the high degree of optical scattering by the skin, which makes it more difficult for optical imaging to penetrate deeply. For example, OCT angiography (OCTA), a functional extension of OCT for visualizing blood vessels, typically cannot be used in skin due to the high degree of scattering within the epidermis and dermis before reaching the deeper vascular layers. But in recent years, considerable research has addressed the challenge of skin imaging, including the introduction of clinical dermatologic OCT systems, the use of OCT for studying derm pathology, and the technical updates needed for further advancements of OCT in dermatology.
OCT in dermatology
OCT found applications in skin imaging and treatment within a few years of its invention1. However, the use of time-domain detection limited the speed, sensitivity, and ultimately the penetration depth that could be achieved until the adoption of Fourier domain OCT techniques in the early 2000s. With improved imaging capabilities, multiple OCT systems for dermatology have come to market (Figure 1).
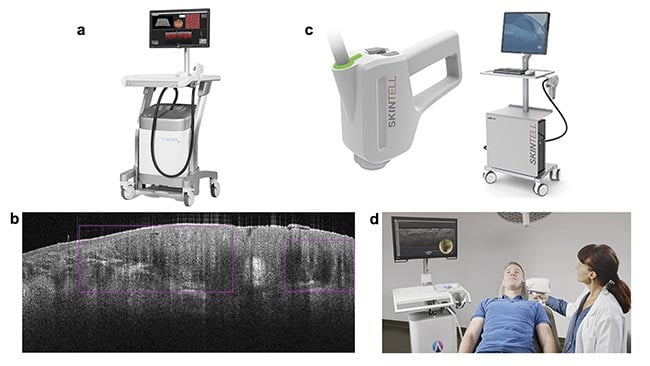
Figure 1. Commercial systems for dermatology using OCT. The VivoSight from Michelson Diagnostics (a). The image marker for
basal cell carcinoma found by AI and the VivoSight (b). Courtesy of Michelson Diagnostics. The Skintell from Agfa (c). Courtesy of
Schlagheck Design GmbH. The deepLive from Damae Medical in use (d). Courtesy of Damae Medical.
The VivoSight from Michelson Diagnostics Ltd. was the first OCT system intended solely for imaging skin and was the first Fourier domain-OCT system to receive FDA clearance via 510(k) (a demonstration that the device is as safe and effective as others currently on the market) for non-ophthalmological application in 2010. The VivoSight device is also in routine clinical use in Europe for differentiating skin lesions. The system uses a swept-source Fourier domain OCT approach to achieve high-resolution measurements over a 6- × 6-mm field of view. The A-line rate of 20 kHz is slightly low compared with the line rate of retinal OCT scanners, but the device leverages a multibeam approach to improve frame rate. The spatial resolution of a few micrometers limits the device from achieving cellular resolution, but the use of a 1300-nm source enables ~1 mm of penetration depth into the dermis.
The Skintell by Agfa was approved by the FDA for commercial sale in 2014. It can be viewed as a higher performance OCT instrument offering 3-μm resolution in both the lateral and axial dimensions, enabling cellular details to be visualized. The A-scan rate is 320 kHz, providing a faster acquisition, but the field of view is smaller at only 1.8 × 1.5 mm. Penetration depth is given as 1 mm in tissue but is described as a factor of two lower than the VivoSight, based on regulatory filings. The Skintell is no longer commercially available.
The most recently cleared OCT device for dermatology is the deepLive from Damae Medical, receiving approval for commercial sales in July 2024 from the FDA (it is also CE marked for sales in European Union countries). It offers the highest resolution of these three examples, with <1.5 μm in both axial and lateral directions by using visible and near-infrared light from a supercontinuum source in a unique line-field configuration. The penetration depth is listed as <0.4 mm with a narrower field of view of 1.2 × 0.5 mm. This device is most well suited for high-resolution imaging of skin cellular structure, which provides vital insights into skin condition and disease.
Examining skin cancer
While several applications of OCT for dermatology have been pursued by researchers and clinicians, including evaluation of psoriasis, wound healing, burn depth, and aesthetics, the primary application in biomedical imaging has been in the evaluation of potentially malignant skin cancer lesions. The deadliest form of skin cancer is melanoma, yet OCT has not shown the diagnostic accuracy needed for this modality to serve as an incisive imaging tool for this disease. A key study from 2015 reported OCT diagnosis of melanoma with a 74% sensitivity and 92% specificity2. Perhaps the greatest limitation of OCT for this application is that the structural and pigmented features that are often used for melanoma diagnosis are often not visible in OCT scans.
Instead, other optical techniques may offer better discrimination. For example, the MelaFind, Nevisense, and DermaSensor devices use spectroscopic approaches coupled with AI analysis. However, these devices yield very low specificity, which can lead to unnecessary biopsies and additional costs due to false positives.
Nonmelanoma skin cancers include basal cell carcinoma and squamous cell carcinoma. Although not as deadly as melanoma, these continue to increase in incidence and require advanced methods for screening and surveillance. It is this application that is the best match for OCT, in which the technology can visualize the disruption in normal skin tissue architecture. A recent meta-analysis suggests that OCT has the necessary performance to be clinically useful for the detection of basal cell carcinoma by comparing the effective sensitivity with the accuracy of visual inspection — with or without dermoscopy4. Several new studies seek to employ line-field confocal OCT for analyzing basal cell carcinoma for the high resolution and throughput of the technique (Figure 2). Pending positive results from ongoing studies to establish OCT for this use case, the adoption of this technology may be on the horizon.
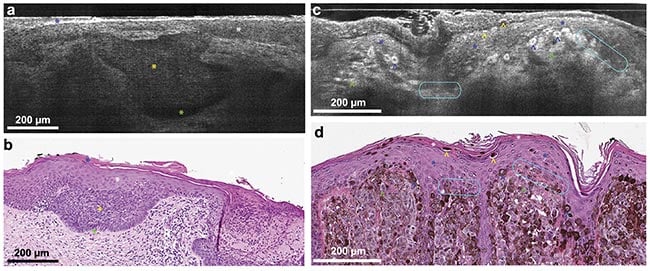
Figure 2. Line-field confocal (LC)-OCT B-scan images of superficial basal cell carcinoma (a) and corresponding hematoxylin and eosin (H&E) stain (b). Blue star: stratum corneum; white star: epidermis; yellow star: clusters of tumor cells that are poorly reflecting in the LC-OCT image; green star: cleft between the tumor islands and the dermis. LC-OCT B-scan images of melanoma (c) and corresponding H&E stain (d). White star: stratum corneum; blue star: epidermis; blue arrowhead: pagetoid spread (epidermal invasion) of epithelial tumor cells;
yellow arrowhead: intracorneal tumor cells in the process of being eliminated; green arrowhead: dermal clusters of melanocytic tumor cells; turquoise circles: partial disruption of the dermal-epidermal junction. Adapted with permission from Reference 3.
Novel approaches
Beyond its potential application in the characterization of skin cancers, novel applications of OCT for dermatology have been developed in recent years. Optical coherence elastography (OCE) employs the high spatial and temporal sensitivity of OCT to quantify the skin’s mechanical properties in response to mechanical perturbations. For example, an air pulse-driven OCE system has been under development for examining sclerotic disease, a plaque-like autoimmune disorder with a high mortality rate (Figure 3). Another approach for OCE is to use ultrasound to perturb the skin and then observe the wave propagation through optical phase analysis in the OCT image. OptoVibronix offers the OptoScope, an integrated OCT/OCE device for characterizing soft materials. OCE approaches can be used to probe the stiffness of various skin layers for the detection of disease, evaluation of therapy, and even for aesthetics.
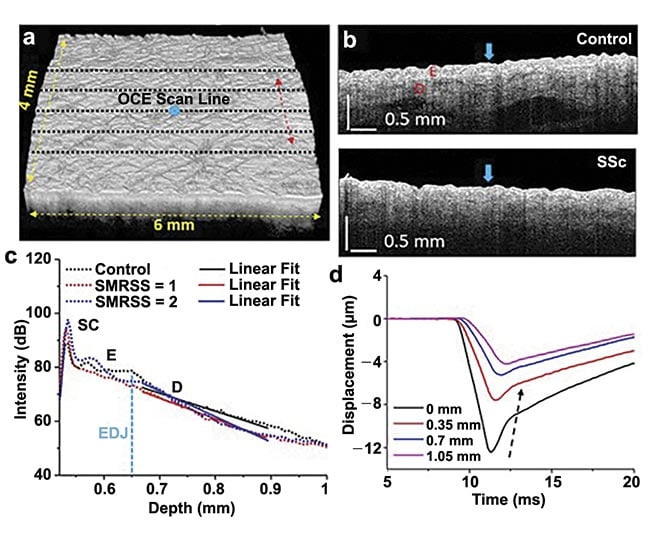
Figure 3. OCT/optical coherence elastography (OCE) imaging of control and sclerotic plaques. A 3D OCT scan co-aligned with OCE scans (black dashed lines, a) and OCT images of typical control and Systemic Sclerosis (SSc) subjects (b), where the air-pulse excitations are indicated by the blue arrows. An example of an OCT A-line with linear fits to obtain the OCT spectral slope in the dermis (c). Temporal displacement profiles of the air-pulse-induced elastic wave propagation, and the black dashed arrow demonstrates the propagation of the elastic wave (d). SMRSS: site-specific modified Rodnan Skin Score. Adapted with permission from Reference 5.
The Department of Biomedical Engineering at Duke University has been advancing OCT for dermatology by introducing new sources of contrast, incorporating spectroscopic information for burn depth assessment, Raman spectroscopy for detection of analytes, and methods for increasing depth penetration6. Figure 4 shows an example of dual-axis OCT where the distinct illumination and detection apertures are used to triangulate a region of interest up to 2.5 mm beneath the skin surface7. Dual-axis OCT has the capacity to image deeper in tissue than single-axis OCT, due to the triangulation of the incident light beam and the detected light path.
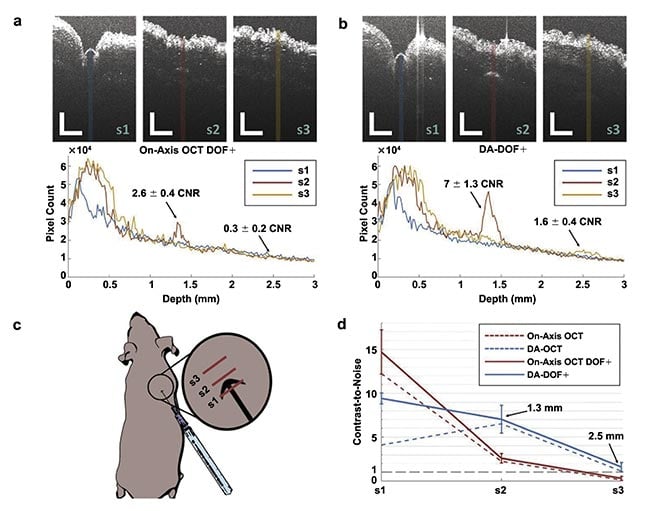
Figure 4. Dual-axis (DA) OCT enables enhanced visualization of a subcutaneous 28-gauge pen needle penetrating mouse skin using two imaging modes: on-axis OCT with enhanced depth of focus (DOF+) (a), and DA-DOF+ (b). Averaged A-line profiles (N = 10) for each image correspond to colored regions of interest shown. OCT scanning orientation (red lines, c). Contrast-to-noise (CNR) analysis for on-axis OCT with DOF+ (red) and DA-DOF+ (blue) concerning sample/needle penetration (d). Red and blue dashed lines represent on-axis OCT and DA-OCT using a fixed focal zone centered at f = 0.75 mm, respectively. The gray line represents CNR = 1. Scale bars: 1 mm. Adapted with permission from Reference 7.
OCT’s future in dermatology
Dermatology has been a compelling target for OCT since its inception. The ability to reconstruct micrometer-scale 3D volumes of tissue structure without taking a physical biopsy seems to be a perfect match for this application. However, the technology has yet to be widely adopted in a clinical setting due to a lack of clear evidence of the necessary sensitivity and specificity for clinical utility. In the field of skin cancer diagnostics, several commercial devices have been released, but it is difficult for these devices to compete with the ease of taking a biopsy and the relatively low cost of tissue processing, which are embedded in the current workflow of dermatologists. In some cases, OCT cannot provide the necessary contrast for analysis, as in melanoma. In other iterations, it might not provide the necessary depth penetration.
High-resolution versions of OCT based on swept source technology produce exquisitely detailed images of tissue structure. However, swept source OCT systems can be expensive and out of the range of many clinical settings, which inhibits their translation to the clinic.
Newer technological developments have introduced new potential use cases for OCT, such as the use of line-field confocal OCT for basal cell carcinoma. Other techniques still in development, such as OCE, may find applications in other areas of dermatology, such as autoimmune disease, in which the body’s immune system attacks healthy skin cells, causing inflammation. As access to OCT continues to expand due to the increased availability of low-cost systems and high-performance systems entering the market — partly due to partnerships between Duke University and Lumedica — greater research efforts will accelerate. Recent advancements in AI for analyzing and interpreting images from a broad database will also likely improve the ability of OCT to detect skin abnormalities.
Meet the authors
Adam Wax is professor of biomedical engineering at Duke University, where he conducts research in development and applications of biophotonics, including clinical applications of OCT. He is founder and president of Lumedica Inc., a manufacturer of low-cost OCT systems; email: [email protected].
David Miller is research scientist in the BIOS laboratory at Duke University. He received his Ph.D. from Northwestern University in 2023. He is also a consultant for Lumedica Inc.; email: [email protected].
References
1. J.M. Schmitt et al. (1995). Subsurface imaging of living skin with optical coherence microscopy. Dermatology, Vol. 191, No. 2, pp. 93-98.
2. T. Gambichler et al. (2015). High-definition optical coherence tomography of melanocytic skin lesions. J Biophotonics, Vol. 8, No. 8, pp. 681-686.
3. A. Dubois et al. (2018). Line-field confocal optical coherence tomography for high-resolution noninvasive imaging of skin tumors. J Biomed Opt, Vol. 23,
No. 10, pp. 1-9.
4. L. Ferrante di Ruffano et al. (2018). Optical coherence tomography for diagnosing skin cancer in adults. Cochrane Database Syst Rev, Vol. 12, No. 12,
p. CD013189.
5. C.H. Liu et al. (2019). Translational optical coherence elastography for assessment of systemic sclerosis. J Biophotonics,
Vol. 12, No. 12, p. e201900236.
6. J. Kim et al. (2015). Functional optical coherence tomography: principles and progress. Phys Med Biol, Vol. 60, No. 10, pp. R211-R237.
7. E.T. Jelly et al (2021). Deep imaging with 1.3 µm dual-axis optical coherence tomography and an enhanced depth of focus. Biomed Opt Express, Vol. 12,
No. 12, pp. 7689-7702.