Commercial thin-film solar technology is making strides in real-world applications, while global research continues to increase efficiency in next-generation thin-film PV cells and coatings.
Solar power is undergoing a strong period of global growth in electricity-generating capacity, driven by declining prices and demand for clean, affordable electricity. In spite of declining government incentives and renewable energy credits, solar photovoltaics (PV) was the most-installed source of new power generation behind natural gas in the U.S. in 2013 and 2014. More than 5.3 GW of new PV systems were installed in the U.S. in 2014, bringing total installed capacity to 16.3 GW1. And this growth should continue around the world for years to come. Solar industry analyst firm Greentech Media in Boston predicts the global cumulative installed solar PV capacity to more than triple from 230 GW in 2015 to 696 GW in 20202.
The vast majority of installed PV systems worldwide are crystalline silicon (c-Si) — wafer-based cells that make up the first generation of solar PV technology. Conventional c-Si technology is time-tested and currently dominates new installed capacity with 90 percent share of the global commercial market. Gallium arsenide (GaAs) and c-Si wafer-based PV technology offer the highest power conversion efficiencies available on the market today, ranging from 15 to 20 percent. Silicon is inexpensive and plentiful, but due to its low absorption coefficient, c-Si must be thick to absorb all available light, which makes it rigid. GaAs is a high-cost material, and wafers must be grown in carefully controlled conditions.
In 1961, researchers William Shockley and Hans Queisser calculated the Shockley-Queisser (S-Q) limit, which predicts the maximum possible solar conversion efficiency of a perfect single junction (p-n) solar cell across the range of semiconductor material bandgaps
(Figure 1)3. According to their calculations, the maximum efficiency of any type of single p-n junction cell is 33.7 percent at a bandgap of 1.34 eV4. The theoretical S-Q limit of nonconcentrating silicon is 29.7 percent power conversion efficiency at a bandgap of 1.1 eV, which means conventional commercial silicon is nearing the practical limit.
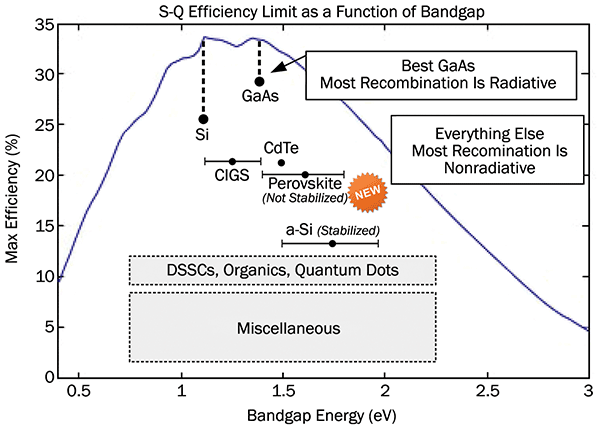
Figure 1. The Shockley-Queisser limit defines the maximum theoretical efficiency of a solar cell with a single p-n junction, according to the bandgap of the material. Si and GaAs currently hold the record for the highest efficiency. CIGS systems are tunable via control of the In/Ga ratio. Photo courtesy of S. Byrnes/Harvard University.
Thin film
Other technologies for which the S-Q limit applies include second-generation cells such as cadmium telluride (CdTe) and copper indium gallium diselenide (CIGS) thin-film solar cells. Thin-film solar cells have historically lower conversion efficiencies than c-Si but are less expensive to produce in volume. They are more efficient at high temperatures and work even on cloudy days. Because they are flexible and lightweight, they can be applied to glass, plastic or metal.
These thin-film PV technologies are commercially available now but claim only a few percent of the global market. What’s promising is that thin film has recently ramped up improvements in efficiency, unlike comparatively stagnant c-Si and multicrystalline Si (µ-Si); see Table 15.
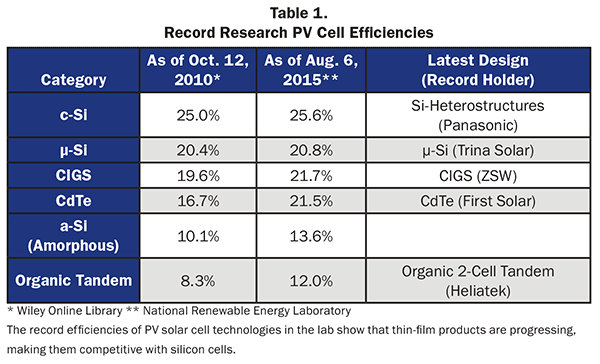
The primary advantage of CdTe thin-film technology over c-Si is its low production cost via common printing or vapor-deposition processes. First Solar Inc. in Tempe, Ariz., has captured approximately 5 percent of the global market for installed PV with its thin-film CdTe technology, primarily in large, utility-scale applications. First Solar holds the current record for the top nonconcentrated thin-film research cell at 21.5 percent efficiency, and module efficiency of 18.6 percent, the latter of which beats the best measured value for µ-Si PERC (passivated emitter rear cell) modules. This makes First Solar’s thin film cost-competitive with first-generation silicon technology. As the S-Q efficiency limit for CdTe is close to 30 percent, the technology may be able to make further efficiency gains in the future.
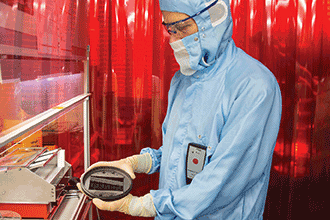
Figure 2. A Sharc25 researcher works in the laboratory with CIGS thin-film solar cells. Photo courtesy of ZSW.
Another commercial thin film, CIGS, has also made marked improvements in efficiency in recent years (Figure 2). The Centre for Solar Energy and Hydrogen Research Baden-Württemberg (ZSW), announced last year a conversion efficiency record of 21.7 percent for a nonconcentrator CIGS solar cell6. In February, ZSW announced another world record efficiency of 21 percent for a close technology cousin — a cadmium-free, small-area CIGS solar cell. The new CIGS architecture deposits intermediate buffer layers of zinc oxysulfide instead of cadmium sulfide to enable higher transmission of blue light into the cell’s absorber layer. The layers are deposited via a chemical bath, which eases its integration into the manufacturing process. The ZSW team and German equipment supplier Manz AG expects the cadmium-free CIGS to reach the market within a few years.
In June, ZSW and a consortium of 10 partners announced a project to push the efficiency of CIGS PV to 25 percent, a goal that would challenge Asian µ-Si dominance, according to ZSW and Empa, the thin film and photovoltaics laboratory at the Swiss Federal Laboratories for Materials Science and Technology (Figure 3). Dubbed Sharc25, for “Super-High-Efficiency CIGS Thin-film Solar Cells Approaching 25%,” the project aims to drop the cost of manufacturing solar modules in Europe to €0.35 per watt peak.
“New insights of the past few years concerning the physics of the CIGS absorber led to an improvement of efficiencies that we would never have expected some years ago,” said Wolfram Witte, Sharc25 project coordinator and CIGS researcher at ZSW.
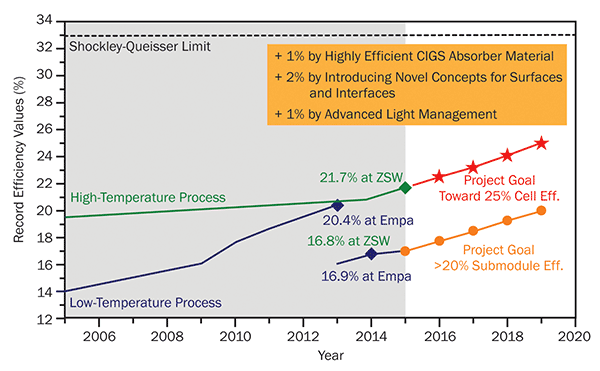
Figure 3. The goals of the European Sharc25 research project consortium include advancing CIGS PV efficiency from its current record value of 21.7 percent to 25 percent. Photo courtesy of Sharc25.
Part of the challenge for CdTe is that the element tellurium is a rare-earth element, rarer than platinum. The amount of tellurium required to provide the world with enough clean energy would eventually be problematic, so it can only be part of the solution. Cadmium is toxic, making it difficult to dispose of. And CIGS involves the use of indium, another rare element. New materials are emerging as viable alternatives, such as copper zinc tin sulphide and combinations of thin film with c-Si.
Third-generation films
The S-Q limit is only valid for semiconductor PV with single p-n junctions. The third generation of PV technology is often defined in the industry as new PV technologies that aspire to overcome the S-Q limit, such as novel semiconductors and emerging thin films.
The top record-holding, research-level cells are multijunction (or tandem) PV cells, which use layers of semiconductor materials with different bandgaps. Spectrolab Inc., a wholly owned subsidiary of The Boeing Co., holds the efficiency record of 38.8 percent in the nonconcentrator, four-junction (or more) category. However, such multijunction cells are quite expensive to produce and are, so far, not commercially competitive with silicon and thin-film technology.
The third generation of photovoltaics include up-and-coming thin-film technologies such as organic photovoltaic (OPV) films, perovskite and dye-sensitized solar cells (DSSCs); see Figure 47. Third-generation PV usually refers to technologies that promise to be even cheaper than second-generation thin-film technologies such as CIGS and CdTe. Third-generation films can be made semitransparent, thin and flexible for application on flat or contoured surfaces such as windows, buildings and even vehicles.
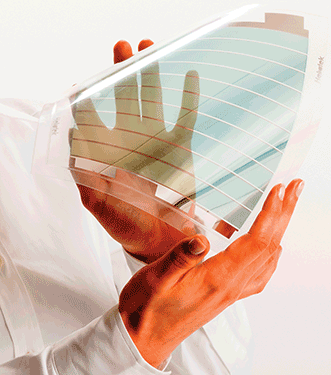
Figure 4. Organic solar film is more flexible than conventional crystalline silicon solar technology and can be transparent. Photo courtesy of Heliatek GmbH.
“The big advantage of these technologies is that they are deposited via liquids instead of vacuum processes, which enables cheap manufacturing at low temperatures,” said Theresa Magorian Friedlmeier, senior researcher in applied physics and materials science at ZSW.
Perovskite is an emerging third-generation thin-film PV technology characterized by a thin crystalline structure that is inexpensive and easy to manufacture. Cell efficiencies have climbed dramatically from 4 percent in 2010 to 20.1 percent in 2014, approaching that of commercial second-generation thin films8. The raw materials for perovskite are abundant, making it promising for large-scale deployment. Furthermore, the S-Q limit for perovskite solar cells is about 31 percent, which makes it attractive for commercial development. Perovskite coatings can be printed onto silicon solar cells, CIGS, cells or glass, delivering a boost to their efficiency. In March, Oxford PV in Oxford, England, a spinoff from the University of Oxford, secured £8 million (about $12.2 million) to continue development of its perovskite PV technology, which can be used in the architectural glass of large buildings to help meet their energy demands.
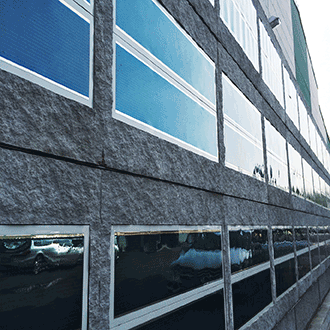
Figure 5. Heliatek organic solar film was successfully integrated with this concrete wall in Herne, Germany, creating a net-zero energy building. Photo courtesy of Heliatek GmbH.
In May, German solar film company Heliatek GmbH, headquartered in Dresden, Germany, announced Europe’s first “solar active” concrete wall at the design headquarters of Reckli GmbH in Herne, Germany. The wall comprises three rows of concrete modules integrated with organic HeliaFilm (Figure 5) that will produce energy for use by Reckli. The solar film area, measuring 16.8 × 3.5 m, provides 1 kWp power and is expected to produce 500 kWh of power per year. The southwest-facing wall will deliver more energy on cloudy days and at high temperatures than conventional PV cells, and is a step toward sustainable, net-zero energy buildings.
“HeliaFilm brings solar energy to potentially any building, no matter where, which orientation or what material it is made of,” said Thibaud Le Séguillon, CEO of Heliatek. “We turn the buildings into a power station: This is key to net-zero energy building. In other words, it will reduce the carbon footprint of these buildings.”
All indications are that thin-film technologies will continue to play a significant part in an upcoming prolonged boom for the PV industry.
References
1. M. Makyhoun, et al. (May 2015). Utility Solar Market Snapshot: Sustained Growth in 2014. Washington, D.C.: Solar Electric Power Association. p. 5. Retrieved from www.solarelectricpower.org/media/322918/solar-market-snapshot-2014.pdf
2. A. James (June 2015). Global PV Demand Outlook 2015-2020. Greentech Media. Retrieved from www.greentechmedia.com/research/report/global-pv-demand-outlook-2015-2020
3. S. Byrne (Feb. 2015). The Shockley-Queisser Limit and Its Discontents. PhD Defense, Harvard. Retrieved from http://sjbyrnes.com/shockley_queisser_talk.pdf
4. L.M. Peter (Apr. 4, 2011). Towards sustainable photovoltaics: the search for new materials. Philosophical Trans A, Vol. 369 (doi: 10.1098/rsta.2010.0348).
5. L. Kazmerski (Aug. 6, 2015). Best Research Solar Cell Efficiencies. National Renewable Energy Laboratory. Retrieved from www.nrel.gov/ncpv/images/efficiency_chart.jpg
6. T.M. Friedlmeier, et al. (2015). Improved Photocurrent in Cu(In,Ga)Se2 Solar Cells: From 20.8% to 21.7% Efficiency with CdS Buffer and 21.0% Cd-Free. IEEE J Photovolt, Vol. 5, pp. 1487-1491 (doi: 10.1109/jphotov.2015.2458039).
7. R. Cho (July 2015). Where is Solar Power Headed? Retrieved from http://phys.org/news/2015-07-solar-power.html
8. Retrieved from www.oxfordpv.com/Technology