Advances in indium antimonide (InSb) infrared detectors, detector cooling and system design have resulted in a new generation of application-specific cameras where either SWIR or broadband response is critical.
ELLIOTT RITTENBERG, LEN KAMLET AND ARNOLD ADAMS, IRCAMERAS LLC
Due to their high thermal sensitivity and favorable atmospheric transmission in the 3- to 5-µm spectral region, cameras based on InSb sensors have been used in traditional applications such as medium- to long-range surveillance, as well as for scientific and research requirements where detection of very small temperature differences or wavelength-specific imaging is beneficial. With recent developments, InSb focal plane arrays (FPAs), when properly passivated, have become a viable option to extend the wavelength response of today’s cameras below 3 µm, into the short-wave infrared (SWIR) spectral region, visible and even ultraviolet wavelength regions. This broadband response provides the ability to solve a variety of challenges with a single instrument, and to build application-specific systems that surpass limitations of other infrared imaging technologies.
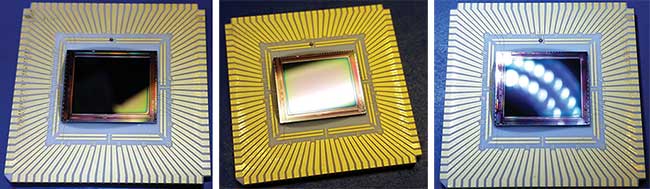
Three passivated but otherwise uncoated InSb focal plane arrays with spectral responses from <200 to >500 nm. FPAs are in leadless chip carriers. Courtesy of IRCameras.
Detector advancements
Indium gallium arsenide (InGaAs) sensors are the most common choice for imaging in the SWIR from 900 to 1700 nm due to their high quantum efficiency, ability to operate at or near room temperature, and relatively low production and packaging costs. “Visible InGaAs” sensors, with a response from 400 to 1700 nm, have also proliferated in recent years. InGaAs sensors, however, have been limited to
an upper wavelength cutoff at 1700 nm due to difficulties in achieving uniform pixel-to-pixel response in two-dimensional
s at longer wavelengths above 1700 nm.
Unfortunately, this leaves these sensors blind in the wavelength region from 1700 to 2500 nm, which contains significant information for both military and civilian applications. Additionally, InGaAs sensors are often subject to image lag or retention when used in very low-light-level applications requiring high-gain states in the sensor.
With advancements in the production of InSb focal planes, infrared cameras today can be equipped with FPAs providing a wide broadband spectral response unparalleled by other production sensors. Advances in the passivation of InSb FPAs eliminate surface states that retain charge when the detector is exposed to higher energy photons, thus removing the possibility of after-images and image lag, which has been an issue for both InSb and InGaAs sensors.
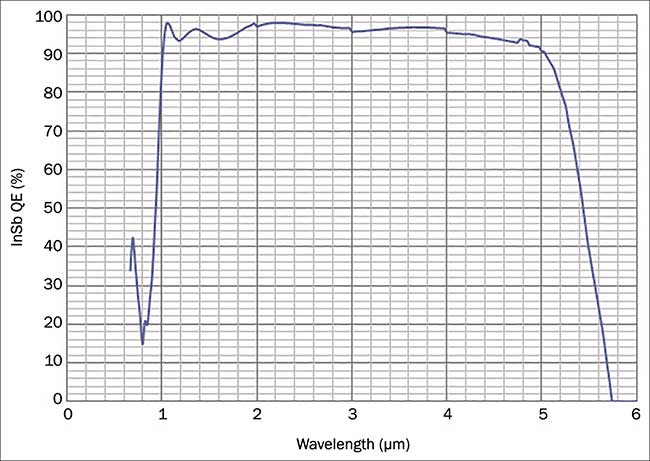
Typical spectral response of InSb FPA with antireflective coating for <1 to >5 μm. Courtesy of IRCameras.
Typically, the quantum efficiency of InSb focal planes prior to the application of an antireflection (AR) coating is about 60% from UV wavelengths to approximately 5000 nm. Improved AR coating processes increase the quantum efficiency to greater than 90% over the wavelength range from 1 to >5 µm. More specialized coatings are available that extend the lower wavelength cut to 400 nm and below, resulting in a single sensor that can be utilized for a broad range of imaging applications.
Finally, the use of digital readout integrated circuits for InSb FPAs results in performance improvements over more traditional analog-based FPAs. Digital InSb FPAs require lower power, eliminate pixel crosstalk, are resistant to blooming and simplify downstream electronics by digitizing data within the FPA instead of requiring an analog-to-digital converter external to the FPA.
InSb sensors with the capabilities
described above are available today in formats ranging from quarter video graphics array (VGA) (320 × 256) to high definition (1280 × 1024), with even higher-resolution (2520 × 2048 and beyond) FPAs just over the horizon.
Cooling InSb sensors
InSb sensors require cryogenic cooling to become photoconductive. Typically, InSb sensors are cooled to about 77 K, though for some applications, including imagers intended for SWIR applications, further cooling of the sensor to less than 70 K is desirable to reduce noise due to dark current.
To cool InSb sensors to the cryogenic temperatures necessary, the focal planes can be integrated into either liquid nitrogen (LN2)-cooled Dewar assemblies, or closed-cycle integrated Dewar cooler assemblies (IDCAs). There are, of course, advantages and drawbacks to each approach.
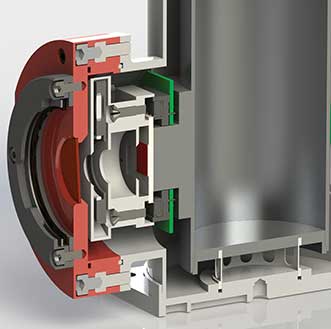
Cut-away view of LN2-cooled Dewar configured with filter wheel. Courtesy of IRCameras.
InSb cameras based on a LN2-cooled Dewar assembly offer the ultimate flexibility for users who may wish to modify the system as application requirements change, or to demonstrate a proof-of-concept before acquiring a closed-cycle cooled system that cannot easily be reconfigured. With an LN2-cooled system, it is possible for the user to easily change cold filters, apertures (ƒ-number) and even the optical back working distance to accommodate a variety of optics, or to define and test the interface to spectrometers and other instruments into which a camera may be integrated. It is also easy to replace FPAs in an LN2-cooled camera to allow upgrades to different format sensors or even different sensor materials.
LN2-cooled systems are also vibration-free, which can be an important consideration when used as an OEM component in a highly sensitive metrology product such as an interferometer. LN2-cooled cameras may also be equipped with motorized multi-position cold filter wheels, allowing the use of spectral filters cooled to cryogenic temperatures to limit their thermal emission and prevent the filter’s emissions from affecting measurements.
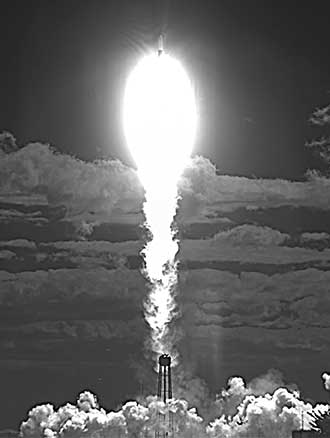
Image of Antares/Cygnus liftoff Sept. 18, 2013, using IRC912 mid-wave infrared camera. Courtesy of MARS Scientific.
The disadvantage of LN2-cooled InSb cameras is that they require replenishment of the LN2, either by filling the Dewar manually, or through the use of a bulky tank and autofill system. For those applications that require cooling below 77 K, the boiling point of LN2, it is possible to lower the temperature of the LN2 by increasing the vacuum above the LN2 reservoir in the Dewar. When doing so, however, the temperature of the LN2 may fluctuate with atmospheric temperature changes or with fluctuating vacuum pressure changes in the liquid nitrogen reservoir, resulting in measurement inconsistencies. For some applications, including astronomical observations, researchers will run InSb sensors at much lower temperatures, below the 63 K freezing point of nitrogen, obviating its use as a cooling medium.
Closed-cycle Stirling coolers provide a method for cooling InSb and other cryogenic cameras designed for continuous operation. These devices remove heat from the detector through the expansion and contraction of helium gas, and are intended for environments where the use of LN2 is not feasible, or for applications where the sensor temperature must be driven below 63 K. Until recently, most cameras so configured incorporated a rotary Stirling cooler, for which manufacturers would historically claim a limited mean time to failure (MTTF) on the order of 8,000 hours. Actual experience, however, has demonstrated this figure to be optimistic. Stirling cooler lifetime is impacted by operating conditions — continuous use in high ambient temperatures and repeated power cycling of the cooler tend to negatively impact MTTF.
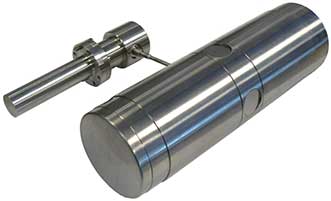
Flexure bearing Stirling cooler. Courtesy of Thales Cryogenics.
Over the past several years, the introduction and maturation of linear Stirling coolers based on flexure bearing technology has resulted in significant improvements in cooler MTTF. Today, a Stirling cooler with a flexure bearing dual opposed piston compressor routinely provides 25,000 hours of operation. Additionally, linear coolers are significantly less prone to vibration than rotary coolers, making the linear cooler a more desirable choice for precision metrology instruments.
An important consideration for IR cameras based on closed-cycle Stirling coolers, whether rotary or linear, is that once the system is built, it is difficult to change its configuration. The detector, cold shield, cold finger and cold filter are housed in a metal Dewar that is welded closed, and must be cut open in order to make changes or effect repairs. Great care must be taken when cutting open a closed-cycle Dewar to ensure that damage is not inflicted on any of the internal components. Also, with the exception of highly specialized custom built systems, a typical closed-cycle IDCA does not support the use of a cold filter wheel assembly due to the increased cooling capacity required by the cold wheel and increased Dewar volume. This limits the spectral response of the system to that defined by the combination of the sensor and cold filter installed in the Dewar. The result is a purpose-built, continuously operable product that can be used either as a stand-alone product, or as an OEM component for integration into an end-user system.
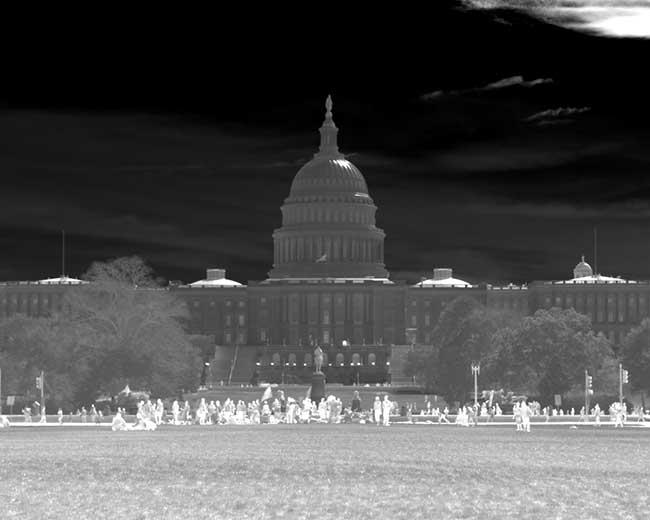
A high-resolution mid-wave infrared indium antimonide image of the National Mall in Washington D.C. with the Capitol building. Courtesy of IR Cameras.
Today’s infrared imaging systems based on high-performance InSb FPAs offer unmatched sensitivity for a variety of application-specific requirements where either SWIR or broadband spectral response is essential. Understanding available options for sensor resolution and coatings that drive the FPA spectral response; cooling technologies and their associated conveniences; optical filters that define system spectral response; and optical designs that describe how the system interacts with the outside world are critical considerations in the selection of these highly enabled SWIR/MWIR imaging cameras.
Meet the authors
Elliott Rittenberg serves as vice president of sales and marketing at IRCameras LLC in Santa Barbara, Calif.; email: [email protected]. Len Kamlet, Ph.D., is product development manager at IRCameras, and has been working exclusively with infrared technology for 14 years; email: [email protected]. Arnold Adams, Ph.D., is chief technical officer at IRCameras; email: [email protected].
Optical Filter Selection
Optical filters are used to define sub-bands within the spectral response of the InSb FPA, and the sub-bands selected are usually specific to a particular imaging application. Filters can be located in the cold space within the IDCA to minimize emissions from the filter itself by cooling it to temperatures close to that of the sensor. This is helpful when looking for small changes in signal that may be affected by contributions from the filter. Conversely, other applications, particularly those where the target is significantly warmer than the background, can make use of room-temperature filters located outside of the Dewar, either between the lens and the Dewar window, or elsewhere in the optical path.
Many applications benefit by limiting the spectral response of the focal plane through the use of optical filters. For long-range surveillance, cameras equipped with 3- to 5-µm cold filters are often employed. However, it is desirable to eliminate the CO2 band, which tends to absorb infrared emissions from a target through the atmosphere. In this case, a CO2 notch filter is involved, which allows transmission from 3.35 to 4.2 µm, and from 4.45 to 5 µm, but blocks signal from 4.2 to 4.45 µm to eliminate the absorption band, which reduces overall signal. In yet another case, cameras designed to detect and image methane and other hydrocarbon gas leaks, a major environmental concern today, are equipped with narrow bandpass cold filters consistent with the absorption bands of these gases.
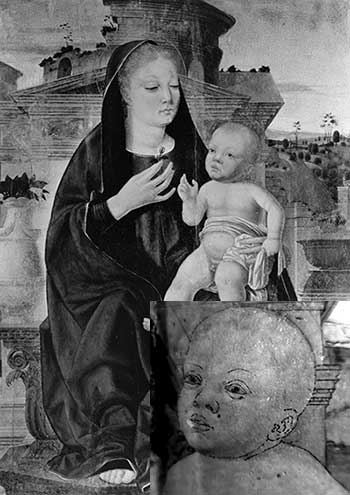
Short-wave infrared reflectograph from a late 15th century Italian painting, ‘Virgin and Child,’ after Francesco del Cossa, shows evidence of the artist’s preparatory drawing under the paint layers. Courtesy of Walters Art Museum, Baltimore.
For many other applications, including spectroscopy, art inspection, hyperspectral imaging, geology and recycling, to name a few, imaging in the 1- to 2.5-µm spectral region is desirable. Using art inspection as one example, scientists and conservators are able to image priceless works of art to look for underdrawings, help determine the nature of the materials used in a given piece, inspect for forgeries and, in some cases, better understand the artist’s intentions by examining revisions made to the painting, which are revealed by imaging subsurface layers. InSb-based systems, with response to 2.5 µm, are useful for this application since the degree of penetration through the various paint layers increases with longer wavelengths beyond the 1.7 µm of InGaAs sensors historically used for this application.
When incorporated as an OEM core into a hyperspectral imaging spectrometer, an InSb camera equipped with a cold filter (enabling 900-to-2500-nm or 400-to-2500-nm response) is suitable for both military and civilian applications. These include detection and classification of chemical threats, mineral mapping, and camouflage detection, as well as agricultural assessments, biomedical screening, pharmaceutical characterization and waste sorting, among many other uses.
Care must be taken when specifying filters for SWIR InSb applications. Efficient transmission through the filter is critical to maintain high sensitivity, as is stray light rejection from longer wavelengths, which can overwhelm SWIR measurements by filling the FPA well with undesired MWIR photons from wavelengths outside those of interest. In some cases, the use of multiple filters in the Dewar has been proven useful to eliminate stray radiation.