Dr. Olga Shenderova, Adámas Nanotechnologies, Inc.
Defect complexes in nanodiamond particles, which consist of vacancies trapped by nitrogen atoms, form different color centers depending on the nitrogen state present in the diamond. New research into these color centers indicates that nanodiamond particles are ideal for molecular-imaging and cell-labeling bioprobe applications.
Nanodiamond (ND) particles have emerged recently as a key platform for many developments in nanoscience and nanotechnology due to their outstanding mechanical performance, biocompatibility and unique optical properties – a combination of assets not often met in the nanoworld. Their superhardness and exceptional chemical resistance motivate the application of nanodiamonds in novel, wear-resistant polymer and metal coatings and as additives in lubricants, where they dramatically decrease friction and wear. The high refractive index and biocompatibility of NDs also make them appealing for use in health care products, such as sunscreens.1
The most exciting area of research has been the unique photoluminescent properties of crystallographic defects in the diamond lattice, which are often referred to as color centers.
Most color centers are based on complexes of vacancies in the diamond lattice in combination with impurity atoms, which can include nitrogen (N), silicon (Si) and certain types of transition metals. The unprecedented photostability of the color centers, biocompatibility and ease of conjugation with biomolecules have encouraged applications using NDs containing color centers as fluorescent biolabels or biomarkers in life sciences.2 Additionally, optically detectable spin properties of selected color centers – particularly the nitrogen vacancy center – have stimulated developments in high-resolution magnetometers, atomic-sized sensors for electric fields, and quantum measurements of temperature gradients within living cells.3,4
Figure 1. (a) A high-resolution transmission electron microscope image of a ~6-nm detonation nanodiamond (ND) particle, demonstrating the perfect crystallographic structure of the diamond nanoparticle core.5 Image courtesy of O. Shenderova et al, reprinted with permission. (b) A schematic model illustrating the structure of a single ~5-nm ND particle after oxidative purification. Structural features of the ND surface include a layer of functional groups (oxygen atoms and hydrocarbon chains are shown in red and green, correspondingly) and patches of graphitic sp2 carbon (shown in black). Image courtesy of Vadym Mochalin, Drexel University.
Nanodiamonds are synthesized by detonation of carbon-containing explosives (called detonation nanodiamonds, as shown in Fig. 1)5 or by fragmentation of micron-sized diamond particles produced by phase transformation of carbon precursors under high pressure and high temperature.1 Nitrogen is the most common impurity in diamond and typically is incorporated into the crystal lattice as isolated, substitutional nitrogen atoms or two nearest-neighbor substitutional nitrogen atoms, among numerous other nitrogen-containing defects. Complexes consisting of vacancies trapped by nitrogen atoms form different color centers, depending on the type of nitrogen state present in the diamond. The nitrogen vacancy (NV) defect (responsible for diamond’s red and near-infrared fluorescence) and nitrogen vacancy nitrogen (NVN) color centers (H3 centers) with bright-green fluorescence are the optically active defects that have received the most consideration.2–4,6 The NV center is a defect formed within the diamond by one substitutional nitrogen atom and a vacancy (Fig. 2), while an NVN center consists of a pair of nitrogen atoms adjacent to a vacancy (Fig. 3). Vacancies can be produced by irradiating the diamond with high-energy particles. High-temperature annealing (greater than about 700 ºC) causes diffusion of vacancies and the formation of complexes with nitrogen atoms.6
Figure 2. (a) Image of fluorescent diamond particles containing nitrogen vacancy (NV) centers captured in an inverted fluorescence microscope. Inset shows the crystallographic structure of the NV center. (b) Photoluminescence emission spectra of 100-nm ND particles containing NV centers dispersed in deionized water at a concentration of 1 mg/ml. Excitation wavelength is 532 nm. Image courtesy of O. Shenderova.
The optical properties of the NV center are well suited for bioimaging applications, with optical excitation ranging from 490 to 560 nm and emission in the red/near-infrared ranging from 635 to 800 nm (Fig. 2a), away from most autofluorescent cell components. Emission occurs in a spectral window of low absorption, which is attractive for biological labeling due to greater penetration of light in surrounding tissue. The spectra of NV centers exhibit a zero-phonon line at 638 nm for the negatively charged NV defect and a zero-phonon line at 575 nm for the neutral state (Fig. 2b). The intensity of the luminescence from NDs containing NV centers depends on the quantity of NV centers within a particle. For example, when compared side by side under identical measurement conditions using total internal reflection fluorescence microscopy, 100-nm NDs may have a photoluminescence brightness exceeding the brightness of the ATTO 532 dye by more than an order of magnitude. The H3 center emits green fluorescence with a maximum intensity around 530 nm when excited by blue light (Fig. 3). A remarkable feature of NV and NVN centers is that they do not photobleach or blink, even when subjected to continuous, high-energy excitation conditions.4 Their unprecedented photostability makes them superior to conventional chromophores.
Figure 3. (a) Image of fluorescent diamond particles containing NVN centers captured in an inverted fluorescence microscope. Inset shows the crystallographic structure of the NVN center. (b) Photoluminescence emission spectra of 70-nm ND particles containing NVN centers dispersed in deionized water at a concentration of 1 mg/ml. Excitation wavelength is 442 nm. Peak at 488 nm corresponds to Raman shift of diamond. Image courtesy of O. Shenderova.
The negatively charged NV center in nanodiamond particles also has emerged as an important element within quantum sensing.3,4 Since the spin state of the negatively charged NV center can be optically detected and the state of the spin is sensitive to the surrounding magnetic field, an ND particle containing a single negatively charged NV center can be used as an ultrasensitive magnetometer working at ambient conditions. Besides magnetic fields, negatively charged NV centers demonstrate high sensitivity to electric fields, temperature and strain.3,4
Depending on the intended applications, ND particles containing either a large number of color centers per particle, or just one negatively charged NV center (one electron spin) per particle, are required. Using ND particles containing a large number of color centers is suitable for applications like biolabels with bright fluorescence. Using ND particles containing only one negatively charged NV center best suits nanosensor applications. Currently, commercially available NDs, ranging from 50 to 100 nm in size, provide bright fluorescence, while particles ranging from 10 to 20 nm in size typically contain one negatively charged NV center per particle (Fig. 4).
Figure 4. (a) Volumetric particle size distribution for NDs with an average particle size of 10 nm and containing an average of one negatively charged NV center per particle and (b) NDs with an average particle size of 100 nm and containing ~900 NV centers per particle. Image courtesy of O. Shenderova.
Since color centers are embedded within the diamond matrix, their fluorescence properties are not affected by surface modification when the centers are located at a distance more than a few nanometers from the surface. Although the surface of bulk diamond is considered chemically inert, nanodiamonds typically contain numerous oxygen-containing, surface-functional groups that are introduced during their purification based upon the use of strong oxidizers.7 Nanodiamonds also can be purified using hydrogen to etch sp2 carbon. Due to multiple methods of surface functionality and, consequently, different surface moieties, nanodiamonds can be readily incorporated into other matrices that are useful for binding biological entities. Such examples include proteins, enzymes, hormones, antigens, DNA or drugs attached via both electrostatic and covalent interactions. A nanodiamond’s surface is amenable to derivatization with a variety of organic functional groups for subsequent linkage with bioactive molecules, making it suitable for use within diagnostic and therapeutic applications.2,7
Nanodiamond particles serve as a platform for several imaging capabilities, as is summarized in Fig. 5. Besides the well-known fluorescence properties of NDs, characteristic Raman signals of pristine NDs were used for the detection and imaging of NDs in biological specimens. Additionally, NDs can be externally labeled with molecular contrast agents for MRI or positron emission tomography.2 The first reported use of fluorescent nanodiamonds (FNDs) containing color centers for in vitro biological labeling appeared in 2005,8 demonstrating that FNDs containing NV centers can be spontaneously internalized by cells while having very low toxicity. The brightness and stability of the fluorescence of these diamonds make them suitable for single-particle tracking within cells. Improved image contrast of nanodiamonds in cells also can be achieved through time-gated detection of the NV center fluorescence, taking advantage of the ND’s long-lived fluorescence lifetime ¬– up to ~20 ns – which is substantially longer than the ~3-ns lifetime for cell and tissue autofluorescence.2
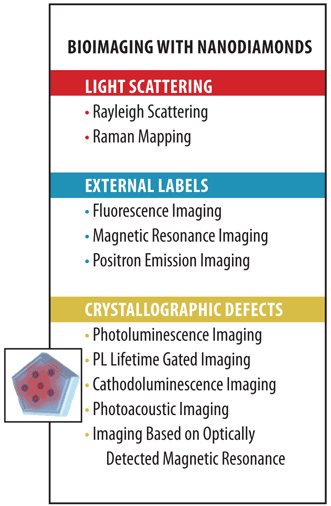
Nanodiamond-based bioimaging capabilities. Image courtesy of O. Shenderova.
The perfect photostability of FNDs enabled superresolution imaging by stimulated emission depletion (STED).9 High-resolution 3-D imaging can be readily achieved in real time. Using STED microscopy, single FND particles (~30 nm) were distinguished in cells with subdiffraction resolution of approximately 40 nm.9
Multiphoton excitation imaging is another powerful tool that allows imaging of living-organism tissues with deeper penetration depths. It excites a fluorophore at the focal spot and thereby reduces cell autofluorescence. Additionally, the multiphoton microscopy provides better image contrast and lower photodamage to cells. The presence of single FND particles (~40 nm) in cells was detected using a femtosecond infrared laser.10
The optically detected magnetic resonance capability of negatively charged NV centers was used to improve the image contrast of FNDs in vitro and
in vivo and to overcome the problem of autofluorescence caused by endogenous molecules. In wide-field fluorescence imaging in vivo, alternating microwave irradiation modulated only the fluorescence intensity of the negatively charged NV center, while background fluorescence remained constant. Image processing effectively removed background autofluorescence signals and significantly improved image contrast.11 In an alternative approach, a modulated magnetic field was used to achieve background-free, wide-field imaging of negatively charged NV centers in NDs, which were located in sentinel lymph nodes.12 The image contrast improved by nearly two orders of magnitude.
Certain color centers in NDs possess cathodoluminescence and remain stable during prolonged electron beam exposure, providing valuable labels for bioimaging based on correlative light and electron microscopy (CLEM). Color images of green and red FNDs within living HeLa cells were obtained using a CLEM microscope,13 revealing structural details with excellent spatial resolution. This demonstrates that FNDs may advance clinical diagnostic sensitivity.
In summary, NDs containing color centers possess bright fluorescence without photobleaching and blinking which, in combination with their biocompatibility and facile biofunctionalization, make them ideal bioprobes for molecular imaging and cell labeling. The unique combination of spin and photoluminescent properties present in certain types of color centers in NDs opens new perspectives for advancing the discovery of new bioimaging and sensing methods.
Meet the author
Dr. Olga Shenderova is president of Adámas Nanotechnologies Inc. in Raleigh, N.C.; email: [email protected].
References
1. V.N. Mochalin et al (2012). The properties and applications of nanodiamond. Nat Nanotechnol, Vol. 7, pp. 11-23.
2. V. Vaijayanthimala et al (2014). Nanodiamond-mediated drug delivery and imaging: challenges and opportunities. Expert Opin Drug Del, Vol. 12, pp. 1-15.
3. R. Schirhagl et al (2014). Nitrogen-vacancy centers in diamond: Nanoscale sensors for physics and biology. Annu Rev Phys Chem, Vol. 65, pp.
83-105.
4. M. Doherty et al (2013). The nitrogen-vacancy colour centre in diamond. Phys Rep, Vol. 528, pp. 1-45.
5. O. Shenderova et al (2011). Surface chemistry and properties of ozone-purified nanodiamonds. J Phys Chem C, Vol. 115, pp. 9827-9837.
6. Y.R. Chang et al (2008). Mass production and dynamic imaging of fluorescent nanodiamonds. Nat Nanotechnol, Vol. 3, p. 284.
7. A. Krueger and D. Lang (2012). Functionality is key: Recent progress in the surface modification of nanodiamond. Adv Funct Mater, Vol. 22, pp. 890-906.
8. S.J. Yu et al (2005). Bright fluorescent nanodiamond: No photobleaching and low cytotoxicity. J Am Chem Soc, Vol. 127, pp. 17604-17605.
9. B.M. Chang et al (2013). Highly fluorescent nanodiamonds protein-functionalized for cell labeling and targeting. Adv Funct Mater, Vol. 23, pp. 5737-5745.
10. Y. Hui et al (2010). Two-photon fluorescence correlation spectroscopy of lipid-encapsulated fluorescent nanodiamonds in living cells. Opt Express, Vol. 18, p. 589.
11. R. Igarashi et al (2012). Real-time background-free selective imaging of fluorescent nanodiamonds in vivo. Nano Lett, Vol. 12, pp. 5726-5732.
12. S.K. Sarkar et al (2014). Wide-field in vivo background-free imaging by selective magnetic modulation of nanodiamond fluorescence. Biomed Opt Express, Vol. 5, pp. 1190-1202.
13. Y. Nawa et al (2014). Multicolor imaging of fluorescent nanodiamonds in living HeLa cells using direct electron-beam excitation. Chem Phys Phys Chem, Vol. 15, pp. 721-726.
Acknowledgment
The author wishes to acknowledge support from the National Institutes of Health under SBIR Contract HHSN268201300030C, NHLBI COAC Services Branch, RFP No. PHS 2013-1, Topic 80, “Fluorescent Nanodiamonds for In Vitro and In Vivo Biological Imaging.”