BALTIMORE, Md., Nov. 20, 2008 – When atoms in a crystal are struck by laser light, their electrons, excited by the light, typically begin moving back and forth together in a regular pattern, resembling nanoscale soldiers marching in a lockstep formation. But, according to a new theory developed by Johns Hopkins researchers, under the right conditions these atoms will rebel against uniformity. Their electrons will begin moving apart and then joining together again repeatedly like lively swing partners on a dance floor.
Moreover, the researchers say, this atomic free-style dancing can be controlled, paving the way for tiny computer components that emit less heat and new sensors to detect bio-hazards and medical conditions.
“By choosing particular atoms in the proper configuration and directing the right laser light at them, we could control the behavior of these ‘nano-dancers,’” said Alexander E. Kaplan, a professor in the Department of Electrical and Computer Engineering in Johns Hopkins’ Whiting School of Engineering. “The essential thing is these are completely designable atomic structures.”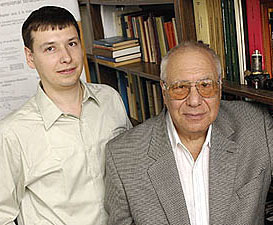
Alexander Kaplan, professor of electrical and computer engineering, and Sergei Volkov, left, a postdoctoral fellow in Kaplan’s lab. Photo courtesy of Will Kirk.
According to Kaplan and Sergei N. Volkov, a postdoctoral fellow in his lab, the next step is for other researchers to conduct lab experiments in an effort to validate the theory and predictions advanced by Kaplan and Volkov.
Kaplan, an internationally renowned nonlinear optics expert who studies how matter interacts with strong light, said his and Volkov’s “nano-riot” idea runs counter to a widely accepted concept. For decades, Kaplan said, scientists have adhered to the Lorentz-Lorenz theory, which asserts that the atomic electrons in a crystal, exposed to a laser beam, will move back and forth in tandem in a uniform way under any conditions.
“But we’ve concluded that under certain circumstances, the nearest atoms will behave much differently,” he said. “Their electrons will move violently apart and come back together again, staging a sort of ‘nano-riot.’”
For this to happen, Kaplan said, several critical conditions must exist. First, the system must be very small, typically involving no more than a few hundred atoms, and the atoms must be arranged in a one-dimensional or two-dimensional configuration. The atoms must be grouped in a sufficiently close concentration; interestingly, though, this arrangement may allow more space between atoms than exists in a typical crystal. Also, the frequency of the laser driving the atoms must be closely tuned to one of the specific frequencies of the atomic electrons – the so-called atomic resonance – in the way that a radio receiver might be tuned to a particular station.
When these critical conditions are met, the interacting excited atomic electrons get strongly “coupled,” and their motion is affected by one another. The atomic dance partners begin to match or counter-match the motion of each other, while still being driven by the laser’s “music.”
When this occurs, the dancing atomic electrons form waves of collective motion. Kaplan calls these waves “locsitons,” based on the words “local” and “exciton,” the latter referring to a physics concept. Within the atomic systems envisioned by Kaplan and Volkov, these locsiton waves are strongly affected by the boundaries of these structures or any irregularities, such as holes. The presence of these boundaries results in size-related resonances, or highly-excited motion at certain frequencies, resembling those of a violin string fixed at two end-points. In this case, the string’s end-points would be the boundaries of the group of atoms. A smooth violin string produces mostly a main tone, and nearby points of the string move in unison. But an atomic array more closely resembles a chain of connected beads, and with the right laser tuning, the neighboring beads, or atomic electrons, can oscillate counter to each other.
“Fortunately, once this atomic structure is built, the ‘dancing style’ of the atoms can be controlled by the laser tuning,” Kaplan said. “Furthermore, if the laser intensity is sufficient, we believe the atoms in this lattice will engage in so-called nonlinear behavior. That means they can be made to behave like switches in a computer. They will act like a computer’s memory or logic components, assuming the positions of either 1 or 0, depending on the initial conditions.”
Computer makers, trying to produce ever smaller metallic or semiconductor components, have run into problems related to the excessive release of heat. However, the nanoscale switch envisioned by Kaplan would be a dielectric, meaning it would involve no exchange of free electrons in the structure. Because of this, the proposed components would generate far less heat.
If their theory is confirmed, the Johns Hopkins researchers foresee other applications for these nanoscale atomic systems. The tiny lattices, they say, could be designed so that when a specific foreign bio-molecule enters a system, the atomic electron ‘dancing’ would stop. Because of this characteristic, they said, the system could be designed to trigger an alarm signal whenever a bio-hazard or perhaps a cancer cell was detected.
The research by Kaplan and Volkov was supported by a grant from the Air Force Office of Scientific Research.
For more information, visit: www.jhu.edu