James Lopez and Yiwei Jia, Olympus America, Inc.
Label-free noninvasive, nondestructive optical microscopy using multiple simultaneous techniques allows researchers to observe diverse life processes in real time.
Optical microscopy is a powerful tool in bioscience research, allowing researchers to observe multiple complex physiological processes in real time. Fluorescence imaging – a major technique used with optical microscope systems – presents many challenges, however. Achieving sufficient concentration of a fluorescent probe within a tissue can be difficult. Phototoxicity caused by singlet oxygen formation is damaging to living cells. And, in live animal and animal tissue imaging, the probe is likely to be recognized as a foreign entity by the animal’s immune system.
Multimodal label-free imaging – including second-harmonic generation (SHG), coherent anti-Stokes Raman scattering (CARS) and intrinsic two-photon excitation fluorescence – offers a technique to monitor disease processes over time without perturbing physiological processes by incision, adding foreign reagents or encountering artifacts caused by fixation, permeabilization or biochemical extraction protocols. There is also an advantage in using label-free imaging techniques based on harmonics or Raman scattering because electrons are not excited in the same manner as in regular fluorescence excitation. In both harmonics and Raman scattering imaging, the formation of reactive oxygen and its subsequent toxicity are significantly reduced.
Multimodal imaging
Multimodal label-free imaging exploits the properties of molecules when they interact with photons from the short-pulsed lasers used in multiphoton microscope systems. These two-photon or multiphoton imaging systems typically are optimized for observation of fluorescent molecules several hundred microns deep in tissue, exploiting the improved penetration and reduced scattering of longer infrared wavelengths. To achieve the “two-photon” effect, these lasers produce an intense flux of photons in femtosecond pulses. The total energy is kept low to avoid overheating the sample.
The only position where the photon flux is strong enough for two or more photons to combine their energies is at the exact focal point. Because there is no excitation outside that spot, fluorescence is confined to molecules in one optical section as the laser scans over the field of view. This inherent optical sectioning also works with other photonic techniques that depend on these photon interactions, including harmonic generation and CARS.
Multimodal imaging is the combination of two-photon techniques such as two-photon excitation fluorescence of intrinsically fluorescent molecules, SHG and CARS in a single experiment. When combined, these techniques create a more complete image of life processes without additional labels.
Most cells and tissues exhibit a complex mixture of spectra from autofluorescence (also called intrinsic fluorescence). It is possible to attribute intrinsic fluorescence of a particular wavelength to molecules such as nicotine adenine dinucleotide (NADH), lipofuscin or elastin. The peak emission for these molecules is in the blue range due to their component aromatic amino acids, so intrinsic fluorescence is often imaged using multiphoton excitation rather than a blue or UV-emitting laser to avoid the photodamage with the higher-energy (shorter wavelength) photons.
SHG occurs when two photons are scattered simultaneously by an orderly array of molecules that all point in the same direction. Known as inversion asymmetry, this type of molecular structure is found in various tissue structures including collagen fibers, myofilaments and microtubules of the mitotic spindle. The second-harmonic signal is emitted coherently – in the direction of the incident beam, with exactly twice the incoming energy and therefore half the wavelength.
In CARS, the three photons that interact originate with two different wavelengths. The difference in frequency gives rise to a signal because of resonance with specific chemical bonds, notably the C-H bond stretch that predominates in lipids.
Methodology
A laser-scanning microscope equipped for multiphoton excitation can be used to image both fluorescence and SHG through the selection of appropriate excitation wavelengths and filters. Intrinsic fluorescence is a hundredfold weaker than most exogenous fluorophores, so the detection path must be optimized by using high-numerical-aperture objective lenses and nondescanned detectors.
SHG signals typically are stronger in the forward direction, so a transmitted nondescanned fluorescence detector and high-numerical-aperture condenser with an oil-top lens is preferred. Forward-directed SHG detection tends to favor large, bright structures, while the reflected direction is often more sensitive to finer, dimmer structures. This is also generally true for CARS imaging. Muscle fibers can be visualized without the use of any labels in Caenorhabditis elegans, a commonly used model organism in biological research, using SHG. SHG and intrinsic two-photon excitation fluorescence (autofluorescence) signals can be collected simultaneously in both the forward and reflected directions, and then can be combined into a single multimodal imaging data set (Figure 1).
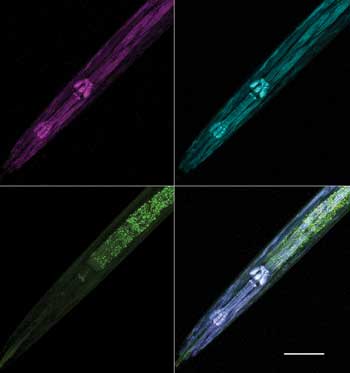
Figure 1. Multimodal imaging of macromolecules in C. elegans. Using multiphoton excitation at 800 nm, a second-harmonic generation signal was collected at 375 to 405 nm in both the reflected (top left) and forward (top right) external nondescanned detectors. Intrinsic two-photon excitation fluorescence (autofluorescence) was simultaneously collected at 520 to 560 nm in the reflected external nondescanned detectors with 800-nm excitation (bottom left, with overlay at bottom right). Maximum intensity projections of 139 Z-series slices acquired at 0.4 µm per optical slice. Scale bar 50 µm. Courtesy of Delong Zhang, Purdue University.
There are several ways to generate the two beams that combine to produce the CARS signal: Researchers have often used two picosecond-pulsed IR lasers tuned to different frequencies to serve as pump and Stokes beams, with the difference in frequency tuned to match the resonant frequency of a specific chemical bond. Recently, however, Adrian Pegoraro et al have developed a femto-CARS system that uses the same femtosecond-pulsed IR laser that is used for multiphoton excitation and splits the beam into two components. One component passes through a photonic crystal fiber to produce a red-shifted Stokes beam, which then recombines with the unaltered beam using precision alignments and stage delay.
The CARS signal can be collected in either the reflected or forward direction with qualitatively different patterns, adding information for expert interpretation. In all detection modes, the IR wavelengths of the imaging beams are blocked, and filters are chosen to separate different signals according to wavelength range. For example, the Olympus femto-CARS system uses two excitation wavelengths for imaging: 800 and 1040 nm. The 800-nm wavelength serves as the imaging wavelength for intrinsic fluorescence and SHG, and is one of the wavelengths for CARS imaging; 1040 nm functions as the second necessary wavelength for CARS imaging. The SHG signal from the 800-nm beam occurs at 400 nm, and the CARS signal is collected at 650 nm.
Depending upon the sample, these three signals (SHG, CARS and intrinsic fluorescence) can be collected simultaneously using either reflected or forward detection.
Additional wavelengths may be used for a fourth signal. A four-channel filter set for the reflected detector might have filters that collect narrow bands for simultaneous imaging of SHG; green fluorescent protein or intrinsic fluorescence; yellow fluorescent protein or the DiI fluorescent indicator; and CARS. Because these imaging modalities are not as specific as antibody- or nucleic-acid-sequence-recognizing probes, most experiments are comparative. For example, the collagen distribution around a tumor may be compared to unaffected stromal tissue or to the pattern observed in a control animal. Researchers might, for example, do a comparison between the intrinsic fluorescence of NADH under normoxic and hypoxic conditions. Metabolism may also be expressed as a ratio of NADH (or NADPH) with another member of the electron transport chain, such as flavin adenine dinucleotide. Similarly, the CARS signal indicates lipids in general but cannot distinguish sphingomyelin from phosphatidylcholine or any other molecule with a long chain of C-H bonds.
SHG imaging of collagen
Collagen deposition around tumors has been recognized by pathologists for decades. Paolo Provenzano et al have proposed a classification of tumor-associated collagen signatures that have potential for clinical staging. These changes in collagen structure can be observed using SHG imaging. Degradation of collagen resulting from extracellular protease activity, as well as changes in fibril composition, may be helpful in identifying changes in tissue that indicate tumor initiation or progression.

Figure 2. Green fluorescent protein expressing tumor growing in liver, imaged using simultaneous multimodal imaging. Collagen fibers (white) are visualized with second-harmonic generation. Lipids are imaged using femto-CARS (red). The GFP-labeled tumor mass and the intrinsic fluorescence are co-observed in the green channel. Maximum intensity projection of a stack of 12 Z-planes spaced at 2.42 µm. Courtesy of Teng-Leong Chew, Northwestern University.
Combined with collagen imaging, CARS imaging of lipids has the potential to help investigate the role of fat-containing adipocytes in the tumor microenvironment. The observation that cancers appear to have an association with fat cells might suggest that adipokines (hormones secreted from adipocytes) promote tumorigenesis by inducing the expression of genes regulating cancer cell proliferation, invasion, survival and angiogenesis (Figure 2). Longitudinal studies may reveal changes in lipid content and fibrosis as the tumor progresses.
Intrinsic fluorescence and CARS
The impact of dietary fats on lipid absorption can be studied using CARS. Individual cells in intestinal microvilli are visualized by their intrinsic fluorescence in the green wavelengths, while the CARS signal, detected in the red wavelengths, highlights lipids both in the lumen of the gut and in microvillus cells (Figures 3 and 4). Such experiments may help in the design of therapeutic interventions for metabolic syndrome and other lipid absorption abnormalities.
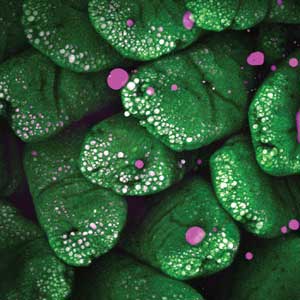
Figure 3. Lipids exterior to and within cells after mice were gavaged with 200 µl of oleic acid. The CARS signal (magenta) from lipids and intrinsic fluorescence (green) delineates individual cells of the microvillus. Maximum intensity projection, 511 Z-planes, 0.5 µm apart, scale bar 100 µm. Courtesy of Mikhail Slipchenko, Purdue University.
More applications of multimodal imaging
In cancer research, recognizing the importance of the tumor cell microenvironment has led to an increase in studies in living animals and animal tissue. Multimodal imaging provides a way to elucidate the relationship between obesity and cancer using CARS imaging of lipids, the mechanisms of invasion and metastasis using SHG, and altered metabolism using intrinsic fluorescence. Epidemiological studies reveal a correlation between certain cancers and obesity, but finding a direct cause-and-effect relationship has proved elusive.
In a series of studies using CARS, Ji-Xin Cheng, Thuc T. Le and colleagues approached this question using CARS imaging of lipids in cells and in animal models. They observed increased lung metastasis and circulating tumor cells in animals fed a high-fat diet. Microscopic imaging of these cells showed increased lipid deposition compared to tumor cells from animals fed a low-fat diet.
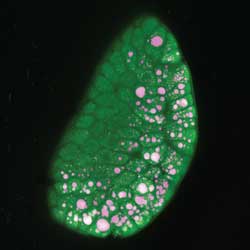
Figure 4. Imaging of lipids within cells of the microvillus, with CARS signal (magenta) from lipids and intrinsic fluorescence (green) delineating individual cells of the microvillus. Maximum intensity projection, 495 Z-planes, 0.3 µm apart with calculated orthogonal slices. Courtesy of Mikhail Slipchenko, Purdue University.
In neuroscience, significant research is being conducted with the ultimate goal of alleviating myelin degradation in spinal cord injury and demyelinating diseases such as multiple sclerosis. CARS imaging of myelin sheath, SHG imaging of astroglial processes and two-photon excitation fluorescence imaging of calcium ion distribution in live spinal tissues may help researchers identify opportunities for intervention.
In animal models of cardiovascular disease, the stability of atherosclerotic plaque can be assessed using multimodal label-free imaging. Lipid-rich macrophages known as foam cells are increased in unstable plaque, while underlying collagen and elastin are disrupted. CARS can identify the lipid content of the accumulated macrophages, while SHG indicates disorganized collagen fibrils; the intrinsic fluorescence of elastin can highlight the vulnerability of the lesion.
Multimodal label-free imaging offers a way to monitor disease processes over time with less physiological perturbation. Now that there are turnkey multimodal systems available, biomedical researchers can combine these methodologies for a more thorough understanding of disease processes. Moreover, these microscopic imaging techniques can pave the way for developing specific molecular imaging techniques that offer greater tissue depth and even the potential for clinical utility.
Meet the authors
James Lopez is the confocal and multiphoton sales application specialist at Olympus America Inc. in Chicago; email: james.lopez@olympus. com. Yiwei Jia is marketing manager of confocal laser scanning microscopes at Olympus America Inc. in Center Valley, Pa.; email: [email protected].
References
K.A. Kasischke et al (July 2, 2004). Neural activity triggers neuronal oxidative metabolism followed by astrocytic glycolysis. Science, pp. 99-103.
T.T. Le et al (January 2009). Coherent anti-Stokes Raman scattering imaging of lipids in cancer metastasis. BMC Cancer, Vol. 9, p. 42.
T.T. Le et al (2010). Shedding new light on lipid biology with coherent anti-Stokes Raman scattering microscopy. J Lipid Res, Vol. 51, p. 3091.
A.F. Pegoraro et al (February 2009). Optimally chirped multimodal CARS microscopy based on a single Ti:sapphire oscillator. Opt Express, Vol. 17, p. 2984.
P.P. Provenzano et al (December 2006). Collagen reorganization at the tumor-stromal interface facilitates local invasion. BMC Med, Vol. 4, p. 38.
P.P. Provenzano et al (2009). Multiphoton microscopy and fluorescence lifetime imaging microscopy (FLIM) to monitor metastasis and the tumor microenvironment. Clin Exp Metastasis, Vol. 26, pp. 357-370.
P.P. Provenzano et al (November 2009). Shining new light on 3D cell motility and the metastatic process. Trends Cell Biology, Vol. 19, pp. 638-648.
P.P. Provenzano et al (December 2009). Matrix density-induced mechanoregulation of breast cell phenotype, signaling and gene expression through a FAK-ERK linkage. Oncogene, Vol. 28, pp. 4326-4343.
M. Skala and N. Ramanujam (2010). Multiphoton redox ratio imaging for metabolic monitoring in vivo. Methods Mol Biol, Vol. 594, pp. 155-162.
M.C. Skala et al (December 2007). In vivo multiphoton microscopy of NADH and FAD redox states, fluorescence lifetimes, and cellular morphology in precancerous epithelia. Proc Natl Acad Sci, Vol. 104, pp. 19494-19499.
This article is the final installment in a series. The first three articles in this series are:
Adrian Pegoraro et al (October 2009). CARS Microscopy Made Simple. BioPhotonics.
Angela Goodacre et al (October 2010). Combining Second-Harmonic Generation
with Multiphoton Imaging. BioPhotonics.
Angela Goodacre and Dennis Donley (September 2011). Intrinsic Fluorescence Lights
Up Cellular Components. BioPhotonics.