L-band tunable lasers with process and performance equivalent to those of established C-band devices create drop-in paired-laser devices with full tunability over 200 consecutive ITU DWDM channels.
David J. Robbins and Andrew J. Ward, Bookham Inc.
The low-loss transmission window for silica fiber lies between 1400 and 1650 nm. Within this region, the range of wavelengths from roughly 1525 to 1565 nm known as the C-band has historically received most attention from telecommunications manufacturers for the dense wavelength division multiplexed (DWDM) systems used in metro and long-haul networks. This is largely because the C-band is well matched to the characteristics of the erbium-doped fiber amplifiers that are the standard technology for providing the optical gain needed to overcome fiber attenuation.
However, there are advantages in being able to use other parts of the transmission window, such as the L-band at roughly 1565 to 1605 nm, either to increase the fiber’s available data-transmission bandwidth or to enable the use of dispersion-shifted fiber for which the C-band is not optimal. Improvements in the performance of erbium-doped fiber amplifiers have expanded their functionality so that they now are routinely available in the L-band.
It is widely accepted that next-generation DWDM communications systems will use full-band — i.e., covering both the C- and the L-bands — tunable lasers to make system planning as simple as possible by maximizing the number of available wavelengths and, hence, ITU DWDM channels. Further, full tunability is essential if dynamic wavelength switching and reconfigurability are to achieve their full potential in the long term.
To ease the transition from fixed-wavelength to fully tunable lasers, manufacturers need widely tunable lasers that are straight drop-in replacements for existing devices in all aspects of performance. Additionally, from a component-manufacturing viewpoint, the elimination of multiple product codes and the simplification of inventory management will significantly lower costs.
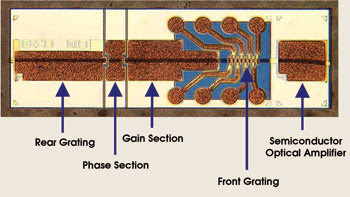
Figure 1. The digital-supermode distributed Bragg reflector laser features four main sections. An integrated semiconductor optical amplifier boosts output and suppresses spurious signals.
Bookham Inc. believes that the best way to achieve these goals is to use a monolithic, InP-based, digital-supermode distributed Bragg reflector laser (Figure 1). The laser features a rear phase-grating section that produces a comb of reflection lines, a phase-control section, a multiple quantum well gain section and a multicontact, chirped grating at the front that is used for coarse wavelength selection. An integrated low-gain semiconductor optical amplifier boosts the output power by as much as several decibels as necessary to provide power leveling across the spectral band. Also, the semiconductor optical amplifier can be reverse-biased to attenuate the laser output and suppress any spurious signals that might interfere with other channels while it is being tuned.
The key to the device’s operation lies in the interaction between the front and rear gratings. Applying an electric current selectively to one or more of the front contacts can shape the front grating’s response (Figure 2). This causes a local change in electron density, which in turn induces a change in refractive index. The shaping of the response can bring the front reflection peak into alignment with one of the rear grating’s reflection peaks. The alignment determines the lasing wavelength by providing the highest optical feedback into the laser cavity.
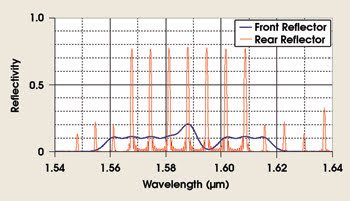
Figure 2. The device lases at the maximum-feedback wavelength, where the peak of the front reflector (blue trace) aligns with one of the peaks of the rear reflector (red trace).
By tuning the front and rear gratings together, the lasing wavelength of the cavity can be tuned over a range on the order of 7 nm, which is the typical spacing of the rear comb peaks. By using each peak in turn and reshaping the front reflector accordingly, it is possible to obtain a quasicontinuous wavelength selection over the full range spanned by the rear reflection comb — typically 45 nm or more. This range is ultimately limited by gain bandwidth roll-off, which leads to competition among potential lasing modes at different wavelengths.
The wavelength range limit approximately matches the wavelength range of the erbium-doped fiber amplifier bands, so it is natural to design the laser to address either the C- or the L-band. Bookham has taken this approach and has produced devices to cover either band.
Module results
Figure 3 shows the power performance of a pair of the digital-supermode distributed Bragg reflector laser modules, one C- and one L-band, power-leveled at +13 dBm in fiber. The semiconductor optical amplifier current required to achieve this power is also shown, typically between 100 and 150 mA. The underlying structure of the tuning mechanism appears as a periodic variation in the current because varying the tuning currents varies the loss in the laser cavity and changes the amplifier gain required to achieve the target power. For similar reasons, the amplifier current is higher for the L-band device, where the nonradiative loss is higher.
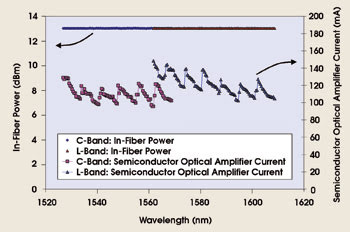
Figure 3. The output of the C- and L-band module is flat between roughly 1525 and 1610 nm (upper trace). The lower trace shows the variation in drive current required to maintain constant optical output at 25 °C. The output shown is provided by two separate lasers, as indicated by the two plots for current.
The devices together yield more than 200 consecutive 50-GHz ITU channels with a total 80-nm range over the C- and L-bands. Typically, in both bands, the side mode suppression ratio exceeds 40 dB, and the linewidth is less than 10 MHz. The relative intensity noise is less than 2140 dB/Hz, and the attenuation using the reverse-biased semiconductor optical amplifier is 30 dB.
The realization of tunable lasers with such attributes is only the first part of creating widespread market acceptance; the lasers must be available in standard electronics/packaging configurations that guarantee performance and calibration to the end user. Compact low-cost transmitters for high-performance metro and long-haul DWDM systems are also needed, simplifying transponder design and minimizing footprint. Components based on digital-supermode distributed Bragg reflector lasers can provide solutions that fulfill these needs and that meet the performance requirements for both traditional metro and 10-Gb/s overlay applications without compromising cost, size or flexibility.
In response to these requirements, the laser has been packaged in higher-functionality subsystems in the same way as for more conventional devices. The discrete laser is used as an in-feed into the Optical Internetworking Forum’s ITLA-MSA standard, which can be used directly or further integrated into MSA-compliant tunable transponders.
Meet the authors
David J. Robbins (e-mail: [email protected]) is a senior technical specialist, and Andrew J. Ward (e-mail: [email protected]) is a senior scientist in the active devices R&D department at Bookham Inc. in Caswell, UK.