Adherence to novel optomechanical design principles can help to create smaller, lighter optical assemblies.
R. Todd Belt and Kevin R. Fine, Agilent Technologies Inc.
The traditional design paradigm that optical and optomechanical engineers use to develop optical assemblies is fairly straightforward and compartmentalized. It starts with the optical design of a series of discrete optics in free space, then involves the design of a metal structure to connect and align them, and finally moves to fabrication and assembly.
With the emergence of better optical production technologies comes a new paradigm: monolithic design, an integrated optical solution in which individual optics directly bond to each other. These designs require few or no metal structures; adhesion and external tooling construct the assembly. However, a successful product requires much tighter integration from the optical designer to the production engineer. Design for manufacturability takes on added importance.
Rules for design
Monolithic design in many ways allows the optimal implementation of various optomechanical and precision engineering principles. These include a mitigation of residual stress and of the mismatch in coefficients of thermal expansion and a reduction in the number of degrees of freedom.
In traditional designs, stresses —such as those produced by preloaded screws, ball contacts and internal dislocations in the substrate — create various problems, including those associated with random relative movement, bulk geometry, birefringence and wavefront. Similarly, as two dissimilar interfaced parts go through thermal cycling, their differential expansion will cause stresses that will lead to misalignment. Monolithic design avoids these issues and further ensures long-term stability by eliminating relative part-to-part movement.
This design paradigm requires a consideration of optomechanical principles specifically related to monolithic optical assemblies:
• Metal still affects design. The assembly eventually will have an interface — specifically, the outer face — that connects to metal. Thus, traditional design rules still apply.
• Use immersed coatings. Monolithic designs often rely on completely contiguous glass-to-glass bonding. This changes the optical and the coating design. The changes in coatings are not more difficult, but it should be recognized that they are different.
• Build on optical surfaces. This is an old principle, but the new twist is that the entire face is used in the bond. This can be very beneficial: One can manufacture higher-precision surfaces onto glass, ceramic and single-crystal than on metal.
• Use computer numerical control manufacturing. Precision is cheaper when obtained from a computer numerical control machine than from manual assembly. This is another old concept, but most designers do not realize that this capability is available in glass, ceramic and single crystal.
• Remove tooling that is built into the assembly. Monolithic optics are aligned during the bonding process. After the bond is stable, the tooling can be removed.
• Rethink failure analysis. Traditional stress analysis is problematic because critical failures in glass, ceramic and single crystal come from random — and therefore unpredictable — local dislocations. Most metals yield at less than 2 percent strain, thus permanently deforming. This can manifest as something as simple as a small dimple from a point contact. Glass, ceramic and single crystal also permanently deform under a 2 percent strain, but the deformation manifests as a break. A good way of improving the validity of the analysis is to ensure that the parts have gone through processes to mitigate subsurface damage, such as thermal annealing, inspection and lapping.
• Avoid brittle critical failure. Most failures occur during handling, so assembly and production teams must pay attention and touch cautiously. In addition, the design should eliminate or mitigate this exposure. For example, avoid cantilevered features and ceramic bolts, and make the outermost interface a ductile material (i.e., metal).
• Beware of the cost of glass, ceramic and single crystal. Parts made with the glass, ceramic and single-crystal method are two to three times more expensive than those made with stainless steel. However, using smaller, lighter assemblies may allow trade-offs in other parts of the system, decreasing overall product cost. There is a balance to be struck between using higher-cost, higher-precision components in the assembly and the elimination of most, if not all, precision metal parts.
• Use adhesion bonding. This is the broadest and most engrossing principle, encompassing optical contacting, low-temperature bonding, room-temperature-vulcanizing sealants, epoxies, soldering and fritting. Some of the advantages over mechanical bonding include no slipping, less machining and lower weight. But the design challenges are adhesion uncertainty, cure time, thickness uniformity, curing-induced stress, coefficient of thermal expansion mismatch, weaker bond, surface finish issues and outgassing.
• Be clean. Consider the example of optical contacting, a surface-energy bond created by ionic, covalent and van der Waals forces. It can be very strong, but only if the distance between the surfaces is on the order of 100 μm or less. Thus, a 100-μm particle can put the bond in jeopardy and cause optical problems. Another example is a doublet. Remember that the optical surfaces are used to properly locate the lenses. In the traditional metal assembly, a few small particles on the outer ring of contact have less effect because of their radial spacing, and they are likely to be compressed, causing an even smaller error. In contrast, these same particles in an epoxied monolithic doublet could be near the center, creating a larger misalignment leverage that compression will not mitigate.
• Integrate design engineering, production engineering and production. Monolithic design requires the cooperative, integrated development of optical, optomechanical and tooling designs. Most of the optical world is not integrated; engineering firms do not have integrated production, and manufacturing firms build to print.
Paradigm in practice
In high-precision applications, monolithic designs are superior because they stiffen the structure and make it more stable. In commercial applications, they are attractive because they enable reduced part counts and less expensive assemblies.
There are immediate advantages to eliminating metal structures. The commercial doublet is an instructive example. Mating two optics using metal parts requires the creation of two surfaces in a tubular housing that must match the tolerances of the optical surfaces (Figure 1 left). The assembly also needs two precision-machined surfaces on the threaded rings to secure the optics in the tubular housing. These essentially are tooling to hold the optics. Tooling works fine for short periods, but the various degrees of freedom make possible long-term drift and hysteresis. It also is expensive to put tooling into a product.
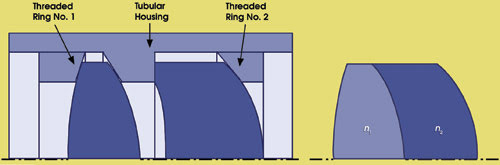
Figure 1. The commercial doublet reveals how eliminating the metal structures from a traditional optomechanical design (left) results in a smaller, less complex assembly (right).
more elegant solution is seen in the monolithic version of the commercial doublet (Figure 1 left). Two facing optical surfaces made of materials with significantly different indices of refraction are designed with the same radius so that the surfaces match and can be bonded. The results of this paradigm are striking in the design of a multiaxis interferometer (Figure 2 left and right). An obvious advantage of the monolithic design is its size. The smaller size and lower part count create other benifits: lower mass, fewer degrees of freedom, higher natural frequency, lower thermal drift, lower thermal drift hysteresis, lower outgassing and tighter tolerances on the assemblies. The dominant glass, ceramic and single-crystal optics also offer inherently excellent material properties.
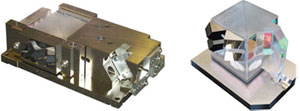
Figure 2. These multiaxis interferometers are similar in that they are both right-turn configurations, but one is a classic metal-and-glass assembly (left) and the other, a monolithic assembly (right). A key configuration difference is that the monolithic interferometer has a larger beam pattern, with three levels and a gap between the first and second levels. A true monolithic equivalent to the classic assembly would be even smaller.
When designing a monolithic assembly, it is critical that the designer think in terms of the new paradigm. Rather than thinking about nuts and bolts, the engineer must consider adhesion and external tooling. Moreover, monolithic design requires close cooperation among all aspects of optical design and production to ensure that the design yields the desired product.
Meet the authors
R. Todd Belt received his bachelor’s degree in aerospace engineering from Arizona State University and his master’s degree in mechanical engineering from San Jose State University. He has worked for Agilent Technologies Inc. of Palo Alto, Calif., since 2001 as an R&D optomechanical engineer; e-mail: [email protected].
Kevin R. Fine received his bachelor’s and master’s degrees from Stanford University with emphasis on precision engineering. He is an optomechanical R&D engineer at Agilent’s Precision Optics and Assemblies Group in Santa Clara, Calif.; e-mail: [email protected].