Molecules Take Step Toward Use as Quantum Bits
GARCHING, Germany, Sept. 25, 2006 -- By creating an optical lattice out of several superimposed laser beams, physicists have succeeded in arranging and keeping molecules in a regular array, an important step toward creating a state in which they could possibly store and exchange quantum information, which is needed if molecules are to be candidates for use as quantum bits, or the memory and processor units of a future quantum computer.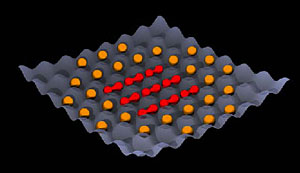
Fig.1: Schematic illustration of the state with one molecule per lattice site. The lattice sites in the center of the lattice are occupied by molecules (red). They were associated from two (identical) atoms using a magnetic field. At the edge of the lattice there are singly-occupied sites with atoms that have no partner to bind with (orange). In the Max Planck Institute of Quantum Optics experiment, the number of (occupied) sites is much larger.
The work was achieved by physicists at the Max Planck Institute of Quantum Optics in Garching, and the resulting shape of the optical lattice resembles a stack of egg cartons, with exactly two atoms placed into the each well of the carton. By applying a magnetic field, these atom pairs are associated to molecules. The work is reported in the Sept. 24 edition of the journal Nature Physics.
In principle, atoms and molecules are equally well suited as memory units for quantum information processing. However, polar molecules, i.e. molecules with a positive and negative charge pole, can interact via electric forces -- very much the way two magnets repel or attract each other depending on their relative orientation. Such an electric interaction is a convenient way for the molecules to communicate with each other and exchange information, and this makes them highly interesting candidates for quantum information processing.
Molecules however, are made up of several atoms, making them complicated objects. While atoms can be cooled down and brought to a standstill using laser light and other tricks, this is not as easily accomplished with molecules due to their complicated internal structure. Their atoms can vibrate against each other and at the same time the whole molecule can also rotate. One prerequisite for using molecules as quantum bits, however, is that their temperature be close to absolute zero, i.e. the molecules should more or less be at rest. The Max Planck physicists also solved this challenge. As professor Gerhard Rempe, institute director, said, “We circumvent this problem by producing the molecules right at the sites of the optical lattice.”
To this end, the researchers transferred a very cold gas of rubidium atoms, a so-called Bose-Einstein condensate, into the optical lattice. The lattice is created by intersecting several laser beams in a certain way. The shape of the resulting light field resembles a stack of egg cartons, where the wells, or lattice sites, correspond to energetically favorable locations. As a consequence, the rubidium atoms prefer to sit at these sites.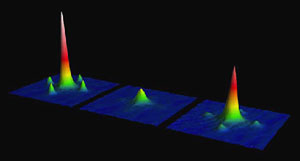
Fig. 2: Interference pictures with atoms and molecules. After switching off the lattice light, the atoms fly apart, which can be observed using a CCD camera. An interference pattern with the typical satellite peaks thereby develops. The three pictures were taken before binding the atoms to the molecules, after binding and after breaking up the bond again. The fact that the first and last of the three pictures are so similar proves that the experiment was successful. The picture in the middle only shows atoms from singly-occupied lattice sites. The molecules are invisible in the detection.
The depth of the lattice sites depends on laser intensity. At low laser intensities, the atoms can move almost freely in the optical lattice and move from site to site. With increasing laser intensities, the wells become deeper and deeper and at some point the atoms cannot escape from the well in which they find themselves -- they are trapped and localized. The resulting highly ordered state is called a Mott insulator state. Changing the overall atom number in the lattice, the physicists were able to create a situation with exactly two atoms per site in the center of the lattice. Only at the edges of the optical lattice atoms were trapped on singly-occupied sites (see Fig. 1).
In the following step, the scientists applied a magnetic field and slowly changed its strength, thus exploiting a phenomenon called Feshbach resonance: Once the magnetic field has reached a certain strength, it becomes more favorable for the atoms in doubly-occupied sites to bind themselves to their partner than to stay single. Those atoms doomed to stay single because they have no partner to associate with are expelled from the optical lattice.
They are of no great use anymore, Rempe said, so “we have ‘blasted away’ the atoms on singly-occupied sites by using other laser light. As a result we got a state with exactly one molecule per lattice site.”
Once the magnetic field is returned to its original value, the molecular bond breaks up again and the atoms return to their initial state. This fact is important for verifying whether or not the experiment has been successfully realized, as only the atoms are visible in the experiment. The molecules are invisible in the detection.
Thomas Volz, who carried out the experiment with his colleagues, said, “Since we broke the bond and could again observe the state with two atoms per lattice site, we made sure that the molecules stayed on their sites and did not escape from the lattice” (see Fig. 2).
The production of a state with exactly one molecule per lattice site is an important prerequisite for the realization of quantum information processing with molecules. The Garching experiment is an important step in this direction, the researchers said.
For more information, visit: www.mpq.mpg.de/mpq.html
Published: September 2006