Boosting MRI signals is helping to secure a place for the technique in visualizing molecular processes in vivo.
Hank Hogan, Contributing Editor
When it comes to peering inside the body, MRI offers some significant advantages over other imaging techniques. It is noninvasive and rapid, does not involve potentially damaging ionizing radiation, and can spot problems deep within tissue. That is why it is the method of choice when athletes, for example, want to know what is wrong with a troublesome knee.
However, when imaging something very small -- like the molecules at the heart of biological processes -- MRI faces some big hurdles. The nuclear magnetic moment that forms the basis for MRI is tiny and, moreover, the molecular sources being observed are not plentiful in the body. So the MRI signal is typically too weak, with the minimum concentration required for detection at least ten -- and possibly as much as a billion -- times off of the micromolar to picomolar concentration range needed.
Now two research groups -- one comprising members from the University of California, Berkeley, and from Lawrence Berkeley National Laboratory and the other at Case Western Reserve University in Cleveland -- have come up with ways to boost that signal, thereby making MRI molecular imaging possible. The eventual result could be a better medical diagnostic tool for detecting such ailments as heart disease and cancer.
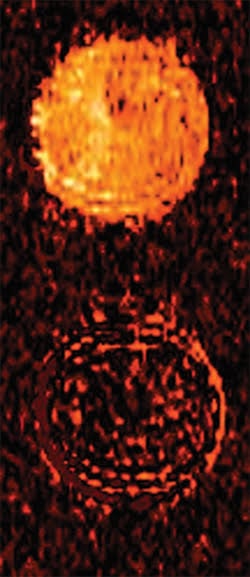
Exploiting biochemistry, researchers at Case Western Reserve University developed a contrast agent for MRI molecular imaging. Before being exposed to a target -- in this case an enzyme -- the signal from a tube is bright (top). After exposure, the signal is weak (bottom), and this effect makes it possible to detect very small concentrations of the target. Courtesy of Marty Pagel.
Hyper imaging
In California, investigators led by Alexander Pines and David E. Wemmer have demonstrated a prototype of a technique that already has the sensitivity needed for molecular imaging. Concentrations in the tens of nanomolars could be easily detectable with reasonable improvements of the group’s current setup, according to team member Tom Lowery.
The technique uses hyperpolarized xenon, which increases the MRI signal 10,000 times. Producing the substance requires exciting a cloud of rubidium atoms with circularly polarized light from a laser operating at 794.7 nm. The nuclear spin of the xenon atoms becomes polarized through collisions with the vaporized rubidium, with as much as half oriented parallel to an external magnetic field and a much smaller fraction antiparallel. Because the signal depends on the difference in orientation, the result is a boost of the MRI peak.
In their work, the researchers used a hollow molecular cage called crypto-phane to hold the xenon, giving rise to a magnetic resonance signal shifted from that of free xenon. To the cryptophane they added a linker and a targeting construct, which can be an antibody or ligand that binds to a specific analyte. This attachment allowed the biosensor to attach to the target and accumulate wherever it exists.
However, rather than detecting the caged biosensor directly, the researchers measured the effect of the biosensor on the free hyperpolarized signal. This effect was amplified because many xenon atoms cycle through the cage in a short amount of time.
Dubbed Hyper-CEST because of its similarity to chemical exchange saturation transfer (CEST), the technique avoids problems that have cropped up. For example, nuclear magnetic resonance frequencies of hyperpolarized xenon are extremely sensitive to the molecular environment but only a few are trapped inside the biosensor, with the signal from the rest effectively wasted. With Hyper-CEST, that is no longer an issue. Different molecules also can be targeted simply by using different biosensors.
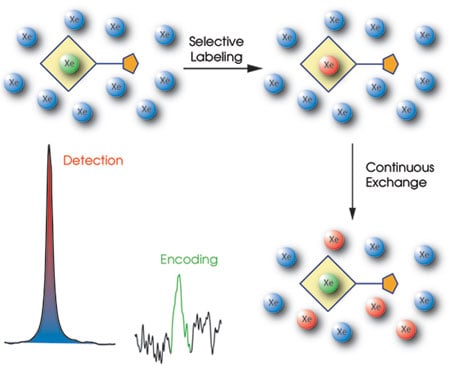
The Hyper-CEST amplification process employs hyperpolarized xenon atoms (blue), using the signal from only biosensor-encapsulated xenon (green) to label the nuclei (red) that pass through the cage. Courtesy of Leif Schröder and Tom Lowery, University of California, Berkeley, and Lawrence Berkeley National Laboratory.
In a demonstration of the technique, the researchers used a biotin-based biosensor and agarose beads functionalized with biotin-loving avidin in solution. According to postdoctoral student Leif Schröder, the bead-based phantom represents cells carrying a target protein.
Free xenon in the bead medium resonated at 193.6 parts per million and free xenon in water resonated at 192.5 ppm. In contrast, the caged xenon biosensor resonated at 65.4 ppm, providing a distinct signal from that of free xenon. This work was published in the Oct. 20 issue of Science.
The investigators next sent a solution containing hyperpolarized xenon through a divided compartment, one part containing biosensor-free beads; the second, the biosensor at a concentration of ~5 μM. Schröder said that these results and others showed that the technique worked. “The specificity is excellent, and the sensitivity is as low as 1 μM for the target protein to be detected.”
Fairly straightforward optimizations would increase the sensitivity sixtyfold, he added, but going beyond that sensitivity would necessitate more complicated schemes, such as having sensors with multiple cages per targeting unit.
In theory the technique could be applied to heart-related diagnostics through the use of biosensors that highlight the plaque associated with blocked arteries. The resulting image would pinpoint accumulated plaque within a patient’s arteries while conventional MRI concurrently mapped blood flow -- the two methods thereby providing a more complete diagnostic.
Before the new technique can be applied to such diagnostics, however, some problems must be overcome. One is a marked decrease (by a factor of 10 or more) in hyperpolarized signal strength that occurs when xenon moves from the lungs into the bloodstream.
The researchers have a number of ideas about how this could be handled. Better models may help show how to optimize the method for different situations, and increasing the hyperpolarization of the atoms could help, as could increasing the occupancy of the cage. Performing the technique on a living organism also will help because the elevated temperature will increase the xenon exchange rate as compared with that at room temperature. That effect will compensate for some of the decreased signal; together with other enhancements, this factor may be enough to solve the problem.
The production of hyperpolarized xenon may have to be streamlined before the technique can be used widely. Better manufacturing methods are the subject of research and, therefore, that issue also may be near resolution.
The researchers plan to repeat the bead experiment in vitro using immobilized cells. If that is successful, they will move to in vivo imaging of a small animal with a solid tumor. Schröder noted that the technique, perhaps in combination with microfluidic devices, could be useful before such studies are done. “The biosensor would not only be valuable for in vivo applications, but also for in vitro diagnostics,” he said.
Multiple contrast agents
Investigators at Case Western Reserve University have demonstrated another indirect technique for MRI-based molecular imaging. In this case, the method starts with a contrast agent of which a fairly high concentration is required for imaging. Enzymes or proteases interact with the contrast agent, resulting in the detection of the target at concentrations as low as a few nanomolars.
Marty D. Pagel, along with graduate student Byunghee Yoo, developed the approach, which is based on the paramagnetic CEST effect. Some of the agents used in this technique respond only to specific environments such as pH, temperature, and the concentration of glucose, lactate, and other biochemicals. However, such techniques require a minimum agent concentration of 1 to 10 mM.
Pagel recalled that he came up with the idea for the indirect approach while driving home from the grocery store. Two papers he had read that week coalesced, giving him the idea of using enzymatic catalysis to change the chemical structure of a paramagnetic CEST agent. That change would shift the magnetic resonance frequencies, thereby lowering the effective detection limit by several orders of magnitude.
In a demonstration of the approach, the scientists designed a contrast agent to detect an active caspase-3 enzyme, which plays a key role in apoptosis and which, therefore, can serve as a biomarker for antitumor therapies.
In constructing the agent, they attached DOTA, a molecule known to be sensitive to paramagnetic CEST, to DEVD, a substrate of caspase-3. Their work was published in the Nov. 1 issue of the Journal of the American Chemical Society.
When scanned from the +100 to –100 parts per million magnetic resonance frequency range, the contrast agent showed a paramagnetic CEST effect at –51 ppm. The researchers attributed this to the amide closest to a lanthanide ion. They then added 48 nM of caspase-3 to the mixture and incubated it for an hour. When they repeated the scan, they found that the signal at –51 ppm was much smaller and that the entire spectrum had changed as a result of a new peak at +8 ppm. This matched the spectrum of DOTA-amine, indicating that the enzyme had converted the amide to an amine. Pagel noted that this was the first reported paramagnetic CEST effect from an amine group, therefore opening opportunities for new MRI contrast agents.
When used in an animal model, the researchers estimated that concentrations as low as a 5.2 mM of the contrast agent can be detected, meaning that detection of caspase-3 enzyme concentrations in the 5 to 50 nM range are possible. The minimum detection threshold will vary, depending on the enzyme’s efficiency in changing the agent’s chemical makeup, the MRI scanner performance and environmental conditions.
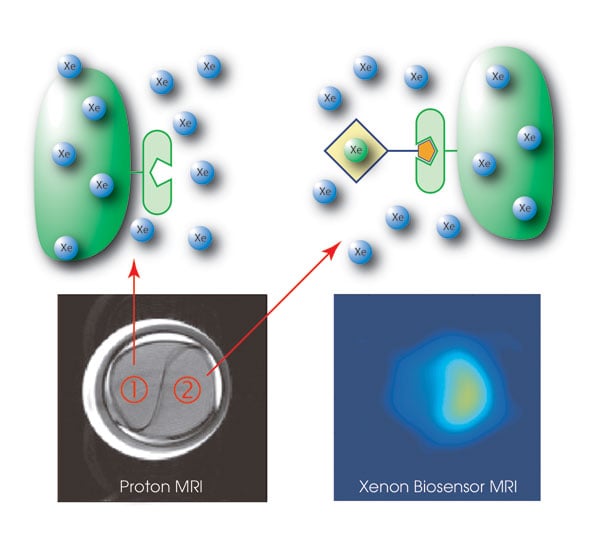
Xenon-enhanced MRI scans highlight a compartment (lower left) that is labeled with a biosensor (2) to track avidin-carrying agarose beads. The biosensor enables molecular imaging at nearly zero background signal (lower right). Courtesy of Leif Schröder and Tom Lowery.
An attractive feature of the paramagnetic CEST effect is that more than one contrast agent can be selectively detected during the same scan, an attribute that can be exploited with the new method in a manner analogous to other multicontrast agent studies. “This technology provides the same advantages of optical dyes -- fluorescence dyes -- that are commonly detected at different optical wavelengths during a single study,” Pagel said.
However, MRI offers good resolution and unlimited tissue penetration depth. As the latter can be a problem for in vivo optical studies, the new technique could enable the imaging of events that heretofore have been shrouded.

The molecular MRI contrast agent consists of a peptide (blue), a magnetic-resonance-sensitive part (red, with a lanthanride ion in purple) and a bond (yellow) that is cleaved by an enzyme. Cleaving causes the MRI signal to change detectably. Using various peptides enables detection of different enzymes and proteases. Courtesy of Marty Pagel.
The scientists have identified 241 proteases besides capsase-3 that work with the technique, leaving them many contrast agents to synthesize and characterize. Synthesizing the demonstration agent was not easy, but they recently developed a solid-phase peptide synthesis method that promises to make the process less challenging. They have a provisional patent on the method and are working with a company to develop it.
The group has already started using the imaging technique in a number of areas. It is developing an agent to track tumor apoptosis in response to photodynamic therapy as well as looking at blood vessels in mouse tumors by using multiple agents with different diameters during the same scan session. In another example, the researchers are using two agents that react to the same compound. One serves as a control for the other, which targets the compound in the tissue of interest.
The goal is to detect two different enzymes during the same scan session, with each offering complementary information about a biological process and not just answering the same question. That work, however, has been held up for reasons that do not entirely involve the contrast agent technology itself. “So far we haven’t found the right application,” Pagel said.