Solar energy piped through fiber bundles helps extract oxygen from the lunar surface.
Kevin Bakhshpour, CeramOptec Industries Inc., and Takashi Nakamura, Physical Sciences Inc.
If NASA has its way, Solar energy and fiber optics will combine to power a high-temperature furnace that mines oxygen on the moon. A manned lunar base will require a substantial supply of oxygen, both for life support and for propellants, and rather than importing it from Earth, NASA is exploring methods for extracting it from lunar regolith via the furnace. The exact composition of the moon’s surface varies with location. Still, aluminum and ferric oxides, feldspars and other silicates abound, although in different proportions. Fortunately, all contain oxygen.
Extracting oxygen from regolith minerals, however, requires substantial amounts of energy. Mineral processing furnaces must achieve at least 1000° C to free the oxygen and up to 1800° C to extract other useful materials, such as iron. Conventional power sources for a system large enough to produce useful quantities are impractical based on the cost of transportation from Earth. Nuclear power is too heavy, and batteries, solar cells and fuel cells have too low an energy density, making them too bulky.
An alternative under investigation is direct use of solar energy to heat materials in the furnace. Physical Sciences Inc., based in Andover, Mass., is developing a solar furnace for materials processing using a mirror system to concentrate sunlight to the energy density needed. The key to keeping the system compact and efficient is optical fiber from CeramOptec Industries Inc. of East Longmeadow, Mass. The fiber will carry the energy from the concentrator to the processing chamber.
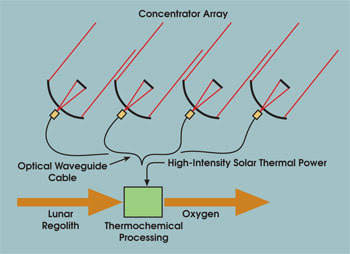
Figure 1. The solar-powered materials processing furnace uses a mirror system to concentrate optical energy into a fiber bundle, delivering as much as 1 kW to the processing chamber.
The solar materials processing furnace (Figure 1) uses banks of mirrors to collect and focus solar energy onto a fiber bundle. The system operates over the 400-nm to 3-μm spectrum, encompassing the majority of the sun’s energy flux. To attain the temperatures required, the concentrators must increase energy density from 3000 to 10,000 times. The furnace design is modular, enabling multiple concentrator arrays and optical energy pipes to be combined to increase energy capacity. The pilot project uses a single unit that can harness 1 kW.
The high-energy density and broad bandwidth place serious demands on the optical fiber cable, which delivers the energy to the reaction chamber. One requirement is a high numerical aperture – at least a 20° half-angle – to simplify coupling energy into the fiber cable. Because the sun is not a point source but a disk 0.4° wide, collimating the concentrated energy is impractical.
The furnace design calls for the fiber cable to be up to 10 m in length, with multiple bends. To keep the size of the concentrator – hence, the launch weight of the system – as small as possible, a highly efficient fiber cable is needed. High efficiency also avoids heat buildup within the pipe.
To address these extreme requirements on the optical fibers, Physical Sciences required fibers that could be formed into bundles to create the optical energy pipe. It used optical fibers that handled energy from 375 nm to 2.4 μm with 80 to 85 percent efficiency, including end losses of 4 to 5 percent. The fibers are made from standard fused-silica core materials and employ a hard polymer cladding, achieving a numerical aperture of 0.48, and the ability to handle an energy density of 1.3 kW/mm2 at 1.06 μm. Drawn from ultralow hydroxyl ion material rods, which were hand-selected for dryness to minimize the fiber’s water absorption peak, the fibers have a 1200-μm-diameter core, 35-μm-thick cladding and a Tefzel outer-jacket diameter of 1400 μm.
The pilot furnace design is under evaluation. The next phase calls for improvements to the optical fiber cable – customizing the preform and rods rather than using stock materials – as well as employing CeramOptec’s PowerLightGuide fused-end bundle assemblies to improve the efficiency of transmission wavelengths.
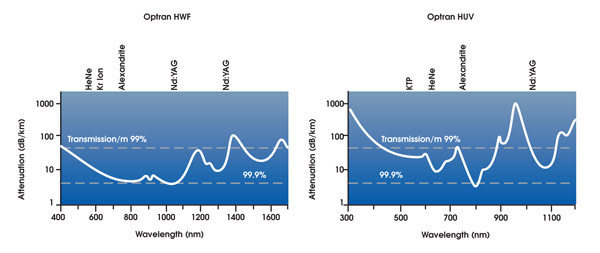
Figure 2. To maximize the optical pipe’s transmission band, the next-generation fiber bundle will use a mix of materials with differing spectral characteristics. HUV and HWF are hard-polymer-clad/pure fused-silica core fibers.
The bundle assembly will contain a mix of high-hydroxyl ion materials for the UV spectrum and low-hydroxyl ion materials for the near-IR range (Figure 2). Adjusting the materials should render the fiber more resistant to radiation-induced darkening, and the numerical aperture should be increased. The fusion process for the fibers inside these bundle assemblies will yield a much higher percentage active area than loose bundling can achieve, enabling greater overall transmission efficiency.
Meet the authors
Kevin Bakhshpour is director of sales at CeramOptec Industries in East Longmeadow, Mass.; e-mail: [email protected].
Takashi Nakamura is general manager of Physical Sciences Inc. in San Ramon, Calif.; e-mail: [email protected].