Machining Advances Lead to Multifunctional Optics
Bernd Reis
Advanced computer numerical control processing allows optics designers
to combine several mechanical and optical functions within one monolithic component.
Today’s optical systems usually serve a variety of functions simultaneously, resulting in ever-more-complex subsystems with increasingly critical requirements related to performance, precision, robustness and miniaturization. More functionality often means more subcomponents,
which limits miniaturization. The solution may lie in combining several mechanical
and/or optical functions within one component to produce multifunctional monolithic
optics (Figure 1).

Figure 1. Compared with a conventional optical
system (top), the benefits of a multifunctional monolithic approach (bottom) could
include using fewer components, minimizing unwanted environmental effects and enhancing
optical parameters.
Overall, advanced computer numerical control manufacturing
systems have enhanced optics production in several ways:
• For conventional optics. Machining
optics in one setup or chucking with a computer numerical control machining system
can reduce scrap rates and processing time, as well as boost productivity and precision.
• For complex 3-D glass and ceramic
components. Computer numerical control machining enables manufacturing of parts
as large as 800 x 800 x 600 mm with micron precision. Production speed and parts
quality also may increase. Building optomechanical systems with components made
from the same or similar material also means that individual components will have
fewer problems related to different rates of thermal expansion.
• For multifunctional and monolithic
optics. Combining optical and mechanical functions within one component enables
designers to miniaturize the optical system without reducing its performance, precision
or robustness. Components also reap the same computer numerical control machining
benefits as the other optics categories. One example is a monolithic prism that
is a central part of a tilt sensor. The prism combines three conventional optical
components: a lens, a rhombus, and a prism- and plane-parallel plate.
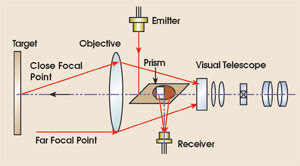
Figure 2. A multifunctional monolithic prism in an electronic-distance-measurement
sensor integrates two rangefinders.
Simplifying optical system design
The goal of designing and building multifunctional
monolithic optics is not only to reduce the number of components, but also to incorporate
overall and concurrent efficiency in design, manufacturing and assembly. For instance,
although the optics’ surface shapes may be very complex, the computer numerical
control machining process can often accommodate these designs without a trade-off
in cost. It also allows designers to minimize or eliminate unwanted environmental
effects that are common to multicomponent systems.
First, take the example of a multifunctional
monolithic scan mirror. Eliminating glued surfaces can reduce adverse temperature
effects on the mirror’s dimensions. Designers often can avoid the effects
of extreme temperature gradients caused by heat expansion or diffusion coefficients
that could lead to temporary or permanent misalignment. Another benefit may be
the elimination of extreme pressure differences caused by outgassings from adhesives
or permeation of liquids into a multicomponent system. Even the aging of adhesives
is no longer an issue.
From a mechanical standpoint, designers
also can eliminate problems related to vibration and shock. There also will be less
risk of unwanted resonance effects compared with a system having a number of components,
each with its own specific resonance frequency.
Now consider the problem of tolerance
stacks common in any multicomponent assembly. In traditional design of such systems,
engineers often must use a variety of techniques to calculate the effect of both
coordinate (positional) and geometric (including mechanical and optical) tolerance
accumulations on individual optical parts and assemblies — their goal being
to ensure proper fit and function among all parts within the assembly. By designing
multifunctional monolithic systems, they can eliminate sources of potential error
during assembly, such as adhesive layers, as well as eliminate or minimize tolerance
stack-up. This can significantly improve the cost-quality relationship.
Optical properties also may improve
with the use of a monolithic optic. In general, when comparing monolithic elements
and classical multicomponent systems, engineers at SwissOptic AG have found that
the monolithic component often has better wavefront properties as well as other
enhanced optical parameters, such as luminosity.
Perhaps one of the major issues driving
the use of multifunctional monolithic optics is the trend toward miniaturization.
Increasingly, OEMs and other end users expect smaller footprints for optical systems
without any compromise in performance. These demands are coming from a variety of
sources, including the sensor, life sciences, information and telecommunications
industries.
For sensor manufacturers, combining
micro-optic elements with optomechanical components offers a new level of integration.
For example, a prism that is a main component of an electronic-distance-measurement
sensor incorporates two rangefinders, including an IR sensor that measures cooperative
targets such as retroreflectors, and a sensor with a collimated diffraction-limited
beam that measures any natural surface, even one with low reflection.
Potential benefits of this design include:
the ability to optimize required interfaces between different subassemblies or materials;
simplified assembly because the alignment and positioning tolerances of the micro-optic
diffractive elements are accounted for during the design stage; and size reduction
— in this case, a tenfold reduction compared with conventional solutions,
because of direct integration of the micro-optic element into the glass prism. The
lens elements are less than 1 mm.
The next step in furthering the integration
level of multifunctional and monolithic optics will involve the use of segmented
dichroic coatings. These would facilitate the inclusion of refractive and diffractive
features, as well as other wavelength-selective functions such as partial mirrors
and filters on one monolithic component. For example, it is now possible to produce
structured optical interference filters with defined patterns of any spectral sensitivity
— on one planar surface (Figure 3). Designers now can structure filters with
a lithographic process on planar surfaces down to less than or equal to 8 x 8 μm
(i.e., high-end CMOS).

Figure 3. With a threefold liftoff coating process, it is possible to produce a red, green, blue filter pattern to a size less than or equal to 8 x 8 μm on a single surface.
Current technology allows structuring any dichroic coating to provide spectral characteristics
specific to each application.
One of the next steps will be to develop
the lithographic process further for the partial coating of etched or replicated
microstructures on the surface of the substrate for spectrally sensitive diffractive
and refractive micro-optics.
Practical considerations
SwissOptic machines multifunctional and monolithic
optics only in glass and does not use a molding process. Therefore, the most significant
cost advantages will come from high-tech applications and lot sizes of less than
50,000 parts per year. When feasible, molding will probably have the advantage in
the serial production of higher volumes.
Meet the author
Bernd Reiss is project manager for process and
product development at SwissOptic AG in Heerbrugg, Switzerland.
Published: September 2002