R. Rüdiger Paschotta, RP Photonics Consulting GmbH
Various gain media used in laser technologies differ greatly in essential properties such as wavelength ranges and tunability, pumping options, efficiency, and capability for energy storage and high powers.
Since the birth of the laser in 1960, a huge variety of gain media have been used: various dielectric crystalline and glass materials, semiconductors, dyes and gases. Although some are definitely more popular than others, a wide range of gain media is still in use, with some strongly differing from one another in many respects. This shows clearly that no single “best” gain medium exists; the choice depends on the application. In each particular case, a large number of properties can be relevant.
Doped insulator gain media
Various dielectric materials have a wide wavelength range in which they are transparent. They can act as hosts for laser-active ions such as Nd3+, Yb3+, Cr2+, Cr3+, Cr4+ or Ti3+, which can be incorporated as dopants. These ions exhibit absorption and laser transitions in certain wavelength regions, which are often – but not always – quite narrow (less than 1 nm) for rare-earth ions and much broader (tens or even hundreds of nanometers) for transition metal ions.
The host medium has a substantial impact, as it interacts with the ions in various ways. The amount of dopant that can be incorporated without problems such as clustering also depends on the host medium. Table 1 gives an overview on common dopant ions, host materials used and accessible wavelengths.
Besides crystalline materials, glasses can be used as hosts, too. Here, the amorphous structure leads to substantially broadened absorbing and laser transitions, but with reduced transition cross sections (Figure 1). An important advantage, particularly for large sizes, is the reduced cost of glasses. On the other hand, the thermal conductivity is usually low, limiting the power handling capability.
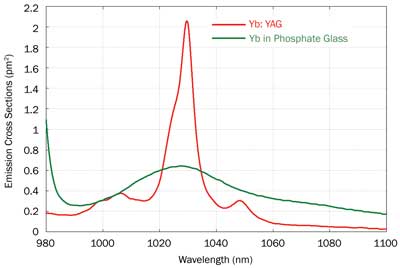
Figure 1. Emission cross sections of crystalline Yb:YAG and of Yb in a phosphate glass. The optical transitions in glasses are generally broader but exhibit lower cross sections.
Optical pumping is the only choice for such gain media. In the early days of lasers, one typically had to use flashlamps and arc lamps, which emit only a small amount of radiation in the wavelength ranges usable for the laser-active ions, but now there are power-efficient laser diodes with an emission well matched to certain pumping transitions. With these, an overall electrical-to-optical power efficiency above 20 percent or even 30 percent can be achieved.
Such diode-pumped solid-state lasers are now used in an enormous range of applications, with output powers from milliwatts to kilowatts and wavelengths from below 400 nm to many microns. With rare-earth-doped glasses and with transition-metal-doped crystals, widely tunable laser operation is possible, although the highest efficiencies and output powers are achieved with media such as Nd:YAG and Yb:YAG, which offer only limited tunability.
The laser transitions of these ions are quasi-forbidden: They have very low transition cross sections but also very long upper-state lifetimes from microseconds to several milliseconds. This provides the opportunity to generate high-energy nanosecond (or sometimes even picosecond) pulses with Q switching: Substantial amounts of energy can be stored in such a gain medium for microseconds or milliseconds and then released within a very short time. The saturation fluence, an important parameter for the design of pulsed laser systems, is rather high. This often indicates the operation of laser crystals with focused beams and very high optical peak intensities.
Ultrashort pulses with picosecond to femtosecond durations can be generated with mode locking, where a substantial emission bandwidth is important, but not the upper-state lifetime. Ti3+:sapphire lasers provide the shortest pulse durations of the order of 5 fs, but many other media, better suited for diode pumping, are used for longer pulses.
Laser crystals and glasses can be fabricated in many forms. Besides the usual cylindrical rods and cuboids, various special forms can be made. As an example, Figure 2 shows a monolithic ring resonator made of Nd:YAG.

Figure 2. An Nd:YAG crystal serves as a monolithic nonplanar ring resonator for a compact single-frequency laser. Laser-active ions, common crystalline host materials and important emission wavelengths.
Rare-earth-doped glasses can also be used for the cores of optical fibers. The small mode areas and long interaction lengths in fibers make it easy to utilize even low-gain laser transitions, so that many more emission wavelengths become available, often with good tunability. Double-clad fibers, pumped with high-power diode lasers, can generate multikilowatt outputs with high beam quality, particularly in amplifier configurations. With chirped-pulse amplification, even rather high peak powers have become possible, despite the high fiber nonlinearity. However, fiber nonlinearities are often a challenge and constitute a limiting factor.
Semiconductors
Semiconductors are utterly different from dielectric media concerning their electronic and optical properties. The relevant electronic wave functions depend on the whole crystal structure and can be influenced strongly by varying chemical compositions; by structures such as very thin layers (quantum wells) and isolated dots of different materials (quantum dots); and by strain resulting from such structures. This opens a huge range of possibilities for engineering semiconductor structures with optimized properties for use as laser gain media in a wide range of wavelengths.
A crucial advantage of semiconductors is their potential for electrical pumping with very high efficiency. Modern high-power laser diodes can reach efficiencies on the order of 70 percent. However, with laser diodes, it is hard to achieve a high beam quality at high output powers. Here, optically pumped lasers with external cavity, pumped with laser diodes, offer interesting opportunities and have started to replace some doped-insulator lasers, particularly where uncommon wavelengths are required.
Compared with the quasi-forbidden transitions of laser-active ions in dielectrics, the optical transitions in semiconductors are very strong, and consequently the carrier lifetime (the analog of the upper-state lifetime) is very short – just a few nanoseconds. This results in a very high gain per unit length, allowing laser operation with a gain medium only a few hundreds of microns long, or even less than 1 µm (in surface-emitting lasers). On the other hand, there is no significant energy storage, so Q-switching and high-energy pulse amplification are impossible.
Wavelength tuning is possible with semiconductor gain media, often in a range of some tens of nanometers. Varying the material composition can often substantially shift that tuning range so that very wide wavelength regions become accessible, although not with a single laser.
Dyes
Laser dyes consist of organic molecules, which in most cases are contained in a liquid solution. (Users often make them by dissolving dye powders in some solvents.) The optical transitions of dyes are relatively broad: tens of nanometers or more – roughly comparable to those of ion-doped glasses – resulting in good wavelength tunability. A big difference between dyes and ion-doped glasses, however, is that these transitions in dyes are not forbidden; they exhibit large transition cross sections, and the upper-state lifetime is correspondingly short (a few nanoseconds). Particularly for pulsed pumping, the gain can easily become rather high, leading to amplified spontaneous emission. The saturation fluence is very low, which implies that high pulse energies can be realized only with very large beam cross sections. Despite the short energy storage time, energetic pulses can be produced if a dye is pumped with a Q-switched laser shortly before it generates or amplifies a pulse itself.
A large number of laser dyes are available, and together they cover huge wavelength regions – the full visible range and also substantial areas in the ultraviolet and infrared region (Figure 3). A dye laser can be operated with various dyes; ideally, different cuvettes and circulation systems are used for different dyes so that an easy change is possible.
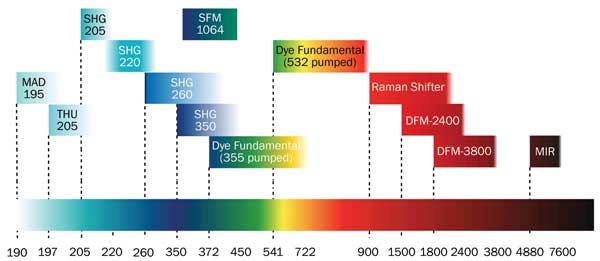
Figure 3. Wavelengths that are accessible with dye lasers and additional nonlinear frequency conversion. Basically, any wavelength in a very wide range can be provided. SFM = sum-frequency mixing; MAD = (nonlinear) mixing after doubling; THU = third-harmonic unit; SHG = single-harmonic generation; DFM = difference-frequency mixing; MIR = mid-infrared.
A notorious problem with dyes is their limited lifetime; they degrade during laser operation. Dye circulation systems are usually required, enabling use of a larger volume of dye solution, which may have a lifetime of a few hundred operation hours. Also, the circulation gives the used dye the time needed to recover from so-called triplet states, where it cannot be used.
The handling of dye solutions can be somewhat tedious and involves various hazards, particularly health hazards resulting from both the dyes and the solvents. Particularly, these substances can be carcinogenic.
Solid dyes would be simpler to use, but their very limited lifetimes and the triplet state problem present serious obstacles for most applications.
Gases
Various gases also can be used as gain media. Although they have some characteristics in common – low densities, high robustness, heat transport by convection, energy input via an electrical discharge, and short (nanosecond) upper-state lifetimes – their properties are very different in some respects.
The first laser gas was a mixture of helium and neon that offers not only the well-known 632.8-nm transition, but also various other transitions in the green, yellow, orange and infrared regions. Laser operation requires only quite moderate current densities but is limited to relatively low output powers.
Carbon dioxide molecules are very different, not only because of the long emission wavelength around 10.6 µm; in CO2, molecular vibrations play a crucial role for the laser process, which occurs at much higher current densities. Output powers can be many kilowatts, and power efficiencies above 10 percent are possible.
Argon-ion lasers (and, similarly, krypton-ion lasers) are also operated at very high power densities. Their efficiencies are far lower than those of CO2 lasers, on the order of 0.1 percent. In earlier times, the low efficiency and limited lifetime of the expensive tubes (a few hundred hours) often had to be accepted, as there was no alternative; for example, for pumping a Ti:sapphire laser. Nowadays, frequency-doubled diode-pumped lasers are often used instead of argon-ion lasers.
Excimer lasers utilize excited gas molecules such as F2, ArF, KrF, XeBr, XeCl and XeF. These rapidly dissociate after laser emission, avoiding reabsorption. Intense ultraviolet laser pulses are obtained for pumping suitable gas mixtures with intense nanosecond current pulses. High-power versions can emit hundreds of watts of average power, which would be hard to obtain with other approaches. Power efficiencies are at most a few percent.
Selecting laser gain media
In many cases, the combination of required emission wavelength and power more or less determines what kind of gain medium is required. For example, carbon dioxide is the only solution for 10.6 µm with high output powers, whereas wavelength-tunable short or ultrashort pulses around 800 nm call for Ti:sapphire.
In other cases, very different approaches may be viable. For example, nanosecond UV pulses around 350 nm can be generated with a xenon fluoride excimer laser, but also with a frequency-tripled Nd:YAG laser. Similarly, tunable output in the visible region may be obtained with a dye laser, but also with a frequency-doubled Ti:sapphire laser or with some kind of optical parametric oscillator or optical parametric amplifier. In many cases such as these, nonlinear frequency conversion provides additional options to reach certain wavelengths. One may then have to compare aspects such as output power and power efficiency, saturation characteristics, cost and complexity, robustness and lifetime.
In the area of doped-insulator lasers, the question “bulk or fiber?” often arises. Although bulk lasers clearly prevail in some areas (e.g., multimillijoule pulse generation) and fiber lasers in others (e.g., diffraction-limited continuous-wave high-power lasers), a wide range of details has to be considered in some areas in order to come to good decisions.
Despite the huge number of gain media available, cases still exist where there is still no satisfactory choice. For example, Ti:sapphire requires a relatively expensive pump source so that directly diode-pumped Yb-doped laser crystals and glasses would present cost-effective alternatives. These, however, are more limited in terms of emission bandwidth and tunability, and sometimes also in terms of output power. Those offering highest output powers often have a more limited emission wavelength range. Broadband gain media, on the other hand, often have a poor power handling capability due to low thermal conductivity, and tentatively lower emission cross sections, leading to low gain and (in mode-locked lasers) to Q-switching instabilities. For these reasons, the search for novel laser crystal and glass materials continues and may bring further substantial advances – even decades after that search began.
Meet the author
Dr. Rüdiger Paschotta is the founder and executive of RP Photonics Consulting GmbH in Bad Dürrheim, Germany, offering technical consultancy and software for laser technology; email: [email protected].