The present-day landscape of lidar is highly competitive and is poised to make a leap forward in cost savings and safety with silicon photonics.
MEHDI ASGHARI, SiLC TECHNOLOGIES INC.
The history of radar (radio detection and ranging) dates back to the 1880s when renowned German physicist Heinrich Hertz showed that radio waves were reflected by metallic objects. Based on the principles demonstrated by Hertz, Christian Hülsmeyer, a German engineer, received a patent in 1906 for radar’s first practical application in maritime navigation. Radar developments then went into full swing prior to WWII for air defense purposes. With advancements in semiconductor technology, radar is now ubiquitous in a multitude of applications ranging from air traffic control to blind-spot detection in cars. Thanks to these advancements, radar technology has made travel by air, land, and water safer and more efficient, and it has prevented hundreds of thousands of accidents and fatalities.
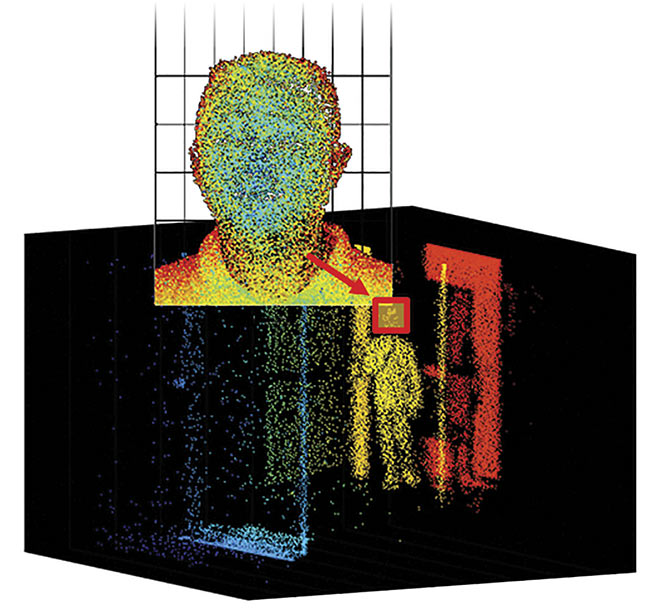
Eye-safe FMCW lidar can be used to provide long-range 3D facial recognition. Courtesy of SiLC Technologies.
Analogous to radar, lidar uses light instead of radio frequency (RF) for detection and ranging. The first lidar systems were realized in the early 1960s, shortly after the emergence of lasers, and could send out a focused light beam and calculate distance by measuring the roundtrip time of the light signal. Since then, lidar has emerged in applications ranging from ground surveying to self-guided autonomous systems (Figure 1).
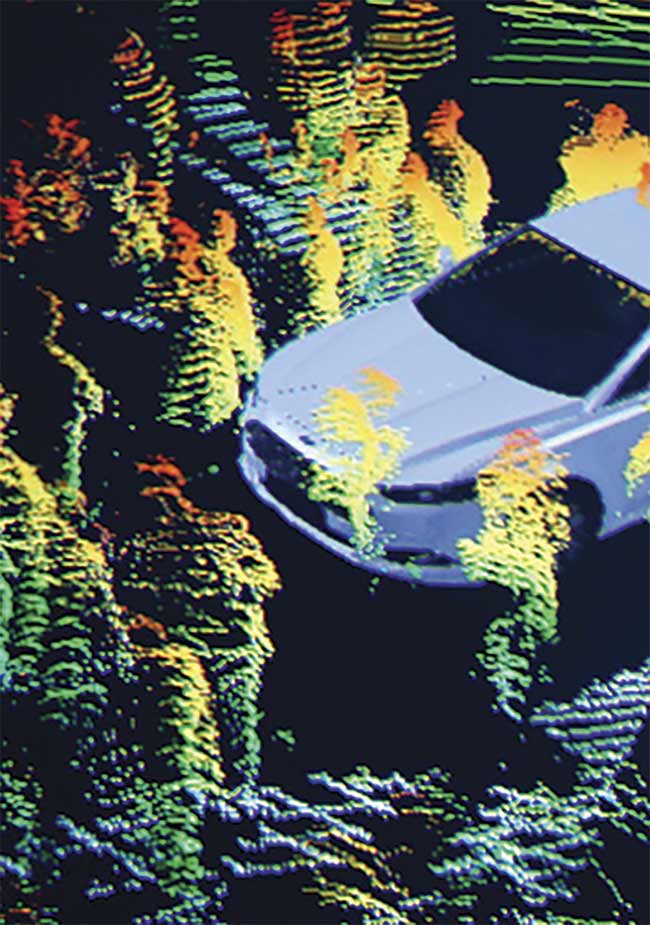
Figure 1. A typical lidar image provides significantly greater detail of the environment. Courtesy of Zuma Press/Alamy Stock Photo.
Radar and lidar
Similar to radar, lidar started out with a simplistic direct-detection approach. A short, bright laser light pulse is fired, and the time it takes for a reflection to return is measured; this determines the distance. This approach is also known as time-of-
flight (TOF) lidar. A scanning lidar sweeps the laser across a field of view and provides a 3D image of the environment. Compared to RF, light — with its much smaller wavelength — has the advantage of a smaller spot size and thus higher resolution.
Radar, however, quickly evolved to use coherent detection methods, which take into account both the frequency and the phase of an emitted signal. The big advantage of coherent radar systems is that small phase shifts of the echo signal are detectable, and the Doppler effect may be used to greatly reduce the influence of fixed clutter or signal from stationary objects. The latest generations of automotive radar have been based on the FMCW (frequency-modulated continuous wave) technique as the optimal modulation for higher transmit efficiency, better sensitivity, and interference tolerance (Figure 2).
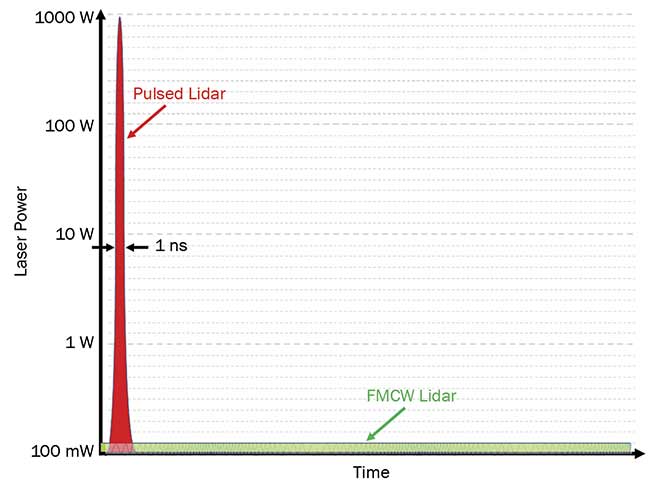
Figure 2. Pulsed lidar functions by emitting a very narrow, high-power laser pulse to measure an object’s distance by measuring the TOF of reflections. In contrast, FMCW lidar sends out low-power frequency chirps. The distance and velocity of the measured object is returned as a frequency shift. Courtesy of SiLC Technologies.
The majority of the commercially available lidar systems are still pulsed lidars because of the lack of availability of two things: inexpensive coherent laser sources and receivers, and associated low-cost chip-integration technologies. Both hamper a transition to the FMCW technique.
Pulsed and FMCW lidars
The pulsed TOF and FMCW approaches are capable of measuring reflections from
highly diffused surfaces such as animals
and clothing. Ultimately, the range and visibility offered by a lidar solution is
determined by the power level and signal-
to-noise ratio (SNR) of the system. FMCW offers >10× better SNR compared to TOF, and its 1550-nm wavelength enables 40× higher power (Figure 3). In the case of pulsed lidar, the SNR, which determines the maximum range, is proportiona1 to the peak laser power1. This requires squeezing all the available photons into a narrow (nanosecond) bright flash of laser light. For 1550 nm, this peak laser power can be as high as a few kilowatts. High levels of peak power, while eye-safe for humans and most animals, are detrimental to imaging sensors.
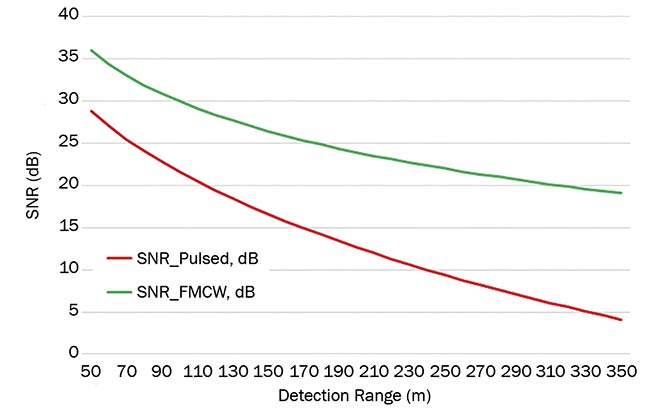
Figure 3. SNR comparison of pulsed (TOF) and FMCW lidar. Assumptions: 1-in. receiver aperture; pulsed lidar uses an avalanche photodiode with a gain of 20, while FMCW lidar uses a simpler PIN photodiode with no gain; target with 5% reflectivity. Implementation loss of 6 dB in both cases. Average laser power of 30 mW. Courtesy of SiLC Technologies.
It’s inevitable that an evolution to coherent detection methods will occur
for lidar as it did for radar. Rather than relying on simple pulses, a few companies are using laser frequency chirps similar
to those already being used in radar systems. These coherent lidars, based on the FMCW technique, allow much higher detection sensitivity and accuracy. They also address many of the shortfalls of direct-detect TOF lidars, such as low immunity to background radiation, multiuser interference, and lack of instantaneous velocity information. For FMCW lidar, the SNR is proportional to the total number of transmitted photons and not to the peak laser power. Furthermore, since FMCW lidar has >10× greater sensitivity, it can transmit at <10× lower average power than pulsed lidar. Hence FMCW lidar can transmit at less than 100 mW of peak laser power (a 104 smaller value) with longer pulses to achieve the same SNR as pulsed lidar, while using less power (Figure 4).
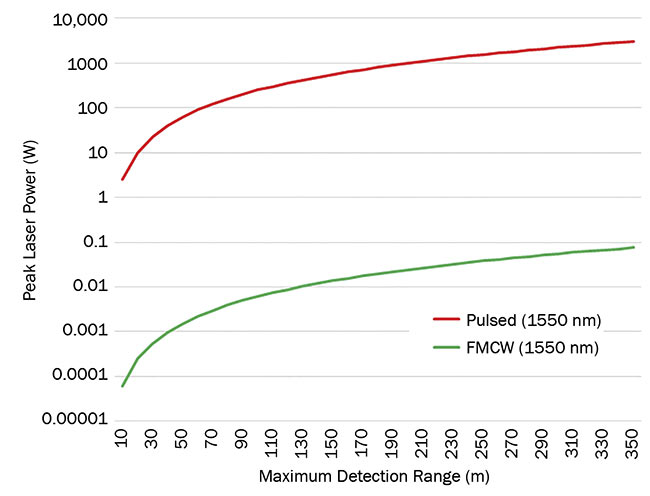
Figure 4. The peak laser power requirement for FMCW compared to pulsed lidar is more than four orders of magnitude lower. Courtesy of SiLC Technologies.
A recent study by Bastion Schwarz and colleagues2 indicates that peak laser power is more detrimental to CMOS/CCD sensors than average laser power. The damage threshold for CMOS and CCD sensors was determined to be 10 kW/mm2. Since a camera lens can even further focus the light, and hence lower this value by a factor of 10,000, potential damage to cameras can occur with as little as 1-W
laser pulses. If just a fraction of this power is necessary, FMCW lidar is inherently more camera- and eye-safe.
Reflectivity and cost
Two strengths of the 1550-nm FMCW lidar systems are reach and instantaneous
velocity data. The combination of higher SNR from coherent detection and higher eye-safety enabled by the 1550-nm wavelength means these lidars can easily detect tires or similar low-reflectivity objects more than 300 m ahead of the car when driving on the highway. This distance allows sufficient time for a vehicle traveling at 70 mph to stop or change lanes in extreme weather conditions, including a heavy storm with lightning. FMCW also enables instantaneous measurement of the velocity of an object via the Doppler effect. This capability is important, not only because it enables predictive analysis and response, but it also enables differentiation of objects from the raw point cloud data.
To date, the key challenge with bringing an FMCW solution to market has been the lack of low-cost, high-volume manufacturing of the required high-
performance components. The coherent
technique requires lasers with long coherence lengths (narrow linewidths) so the solution can work for spans of up to
300 m. The FMCW solution also requires coherent processing of the light to extract additional information carried by photons. This means very accurate, low-noise optical signal processing (OSP) circuits are needed to form a coherent receiver. In addition, polarization plays a role here because coherent mixing will only work for photons of the same polarization. Furthermore, wavelength stability and linearity of the laser source is critical over the measurement periods; otherwise, the SNR can be degraded significantly.
Creating such a stable, robust, and
accurately defined optical system out of
discrete components is difficult and expensive. Two startups, Blackmore Sensors and Analytics Inc. (acquired by Aurora in May) and Aeva Inc., were selected by two prominent German carmakers and received significant funding to work on the FMCW solution. Existing systems based on telecom-grade components can cost tens of thousands of dollars. This price tag might be affordable for use in commercial autonomous cars that replace a human driver and operate 24/7, but not for the mainstream consumer vehicle with a level 3 or 4 advanced driver-assistance system. There is hope that advancements in semiconductor technology will eventually bring down the price of lidar.
Silicon photonics
The good news is that the same technology that enabled the integration of complex electronic circuits into small silicon chips (which are now ubiquitous within household consumer products) has an optical equivalent — silicon photonics. Silicon photonics enables highly complex optical circuits to be cost-effectively manufactured and integrated into an equally wide range of products and applications.
To enable affordable FMCW lidar solutions, all optical functionalities need to be integrated into a single silicon chip that incorporates the same semiconductor processes used to manufacture nearly a trillion electronic integrated circuit (IC) chips every year.
The key challenge to bringing an FMCW solution to market has been the lack of low-cost, high-volume manufacturing of the required high-performance components.
Such a technique promises to offer the level of manufacturing control and accuracy needed for integrated circuits to achieve superior performance, and the functionality needed to enable a robust, cost-effective, and high-quality product. An extremely low-noise, low-loss, and polarization-independent technology will enable very high SNRs, so even lower optical powers can be used. Thus, even when humans are close to the sensor, it will remain eye-safe for detection of distant objects. Measuring objects a few centimeters in dimension >200 m away should be within the capability of integrated circuit-based FMCW lidar. Such a solution was demonstrated outside of university research, by SiLC Technologies Inc., in January. The company is now focusing on optimizing the functionality and achieving higher levels of integration.
Silicon photonic circuits, however, are not easy to design. Although they run on standard IC manufacturing process flows, they require a combination of proprietary processes, specialized skill sets, and
expertise for layout and validation. Luckily, they can leverage the more than a billion dollars of investments made in telecom and data center applications over the past decade.
Given their small size, power, and cost, silicon photonic circuits could also be widely deployed in drones and robotics
applications. The circuits need only milliwatts of output optical power, which makes them highly power-efficient.
Efficiency can be important for battery-operated machines. Silicon photonic circuits can achieve high-precision levels in the millimeter-to-micrometer range, which also enables potential biometric, industrial, or health care applications.
In summary, FMCW solutions transmit at more than four orders of magnitude less peak laser power than current pulsed lidar solutions, while providing improved range, instantaneous velocity, ambient rejection, multiuser interference-free operation, and the potential to integrate the full lidar optical engine into a very small, low-cost silicon photonic chip. These capabilities can transform the use of lidar and enable its broad usage across a multitude of applications well beyond self-driving cars.
Meet the author
Mehdi Asghari is the founder and CEO of SiLC Technologies Inc. He has more than 20 years of experience leading successful photonics companies. Asghari has authored or co-authored over 150 journal and conference publications, and holds more than 40 active patents within the fields of silicon photonics and optoelectronics; email: [email protected].
References
1. T. Fersch et al. (2017). Challenges in miniaturized automotive long-range LiDAR system design, in Three-Dimensional Imaging, Visualization, and Display. Proc SPIE, Vol. 10219, https://doi.org/10.1117/12.2260894.
2. B. Schwarz et al. (2016). Laser-induced damage threshold of camera sensors and micro-opto-electro-mechanical systems,
in Electro-Optical and Infrared Systems:
Technology and Applications XIII; 99870D. Proc SPIE, Vol. 9987, https://doi.org/10.1117/12.2241057.
Lidar Reflectance
The reflectance of surroundings matters, and quoting lidar range performance for high-reflectance targets can be misleading. Therefore, it is important to include a variety of targets in discussions on range performance.
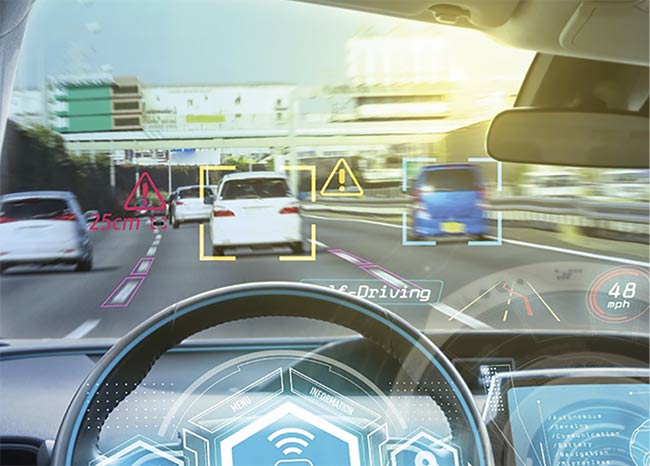
A black tire on the road has a reflectance of 5% to 10%. Courtesy of SiLC Technologies and metamorworks.

(left) A person in a dark hoodie walking at night is nearly impossible to see with either the visible eye or a camera. Textiles (cotton and polyester, wet or dry, 905 nm or 1550 nm) have a lidar reflectance in the range of 30% to 60%. Courtesy of joseelias/123RF.com. (right) Wildlife is another common road hazard. The lidar reflectance of animal fur ranges from 40% to 90%, depending on color and wavelength. Courtesy of welcomia/123RF.com.