Recent developments in Pockels cells and Faraday isolators are paving the way to increased power and higher repetition rates.
MARKUS FEGELEIN, QIOPTIQ PHOTONICS GMBH & CO. KG, AN EXCELITAS TECHNOLOGIES COMPANY
Ultrafast lasers or ultrashort pulse lasers emit pulses in the range of a few femtoseconds up to a few picoseconds. These lasers are essential tools for a variety of applications like laser material processing including cutting, marking, drilling, additive manufacturing and spectroscopic research.
In laser material processing, ultrashort pulses are popular because they only evaporate the material at the laser beam focus position without overheating the surrounding material. Other material processing applications use the high intensity of amplified ultrashort pulses to excite two-photon absorption processes, such as laser cutting of glass, sapphire or cornea — transparent materials that do not absorb the fundamental wavelength but the second harmonic wavelength.
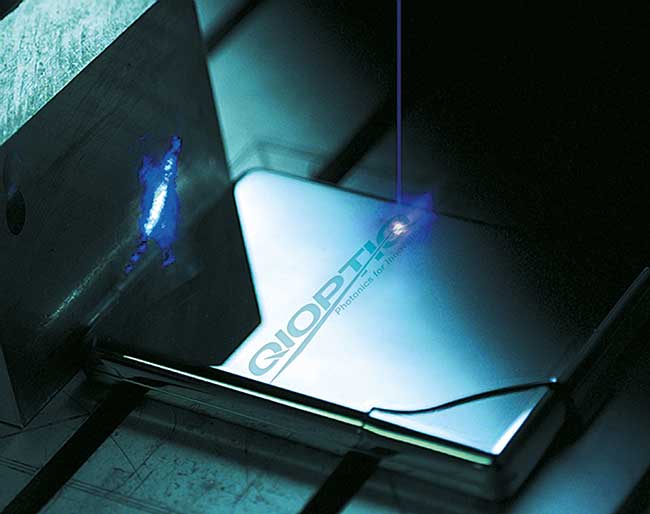
Femtosecond lasers are essential in laser material processing.
Spectroscopic applications make use of the same two characteristics but in a different way. In analog photography, a light flash or a very short shutter time reduces film exposure to freeze fast-moving objects. Similarly, ultrashort laser pulses can be considered ultrafast stroboscopes to observe chemical reactions in the femtosecond time frames where they occur.
The broad wavelength spectrum of ultrashort pulses is a key consideration when designing ultrafast lasers. Short pulses are composed of many wavelengths, the more colors contributing to the pulse, the shorter the pulse can be. Therefore every component that is used in an ultrafast laser’s beam path must be suitable for operation with a broad spectral range. So not only are mirror and window coatings needed to cover a broad spectral range, but the laser media must also emit and amplify over a broad spectral range (Figure 1).

Figure 1. Spectral width of a 400-fs pulse emitted from a Yb:YVO Laser. FWHM: full width half medium. Courtesy of Spectra Physics, High Q Laser GmbH, Rankweil, Austria.
Dopant determines spectral range
The active laser media is the key element of every laser. For solid-state lasers, this most often consists of a glass or crystalline host to which a dopant is added. This dopant roughly determines the center wavelength of a laser while the combination of dopant and host sets the spectral range of the emission, the laser gain itself and ultimately the length of the laser pulse.
Of all common dopants, titanium, hosted in a sapphire crystal, has the broadest amplification spectrum, and therefore is perfectly suited for the generation of pulses in the range of a few tens of femtoseconds.
For industrial applications, ytterbium (Yb) is commonly used since its pump absorption spectrum is very broad and it efficiently converts pump energy into laser light. When hosted in YAG crystals, the gain is so high that the laser rod can be reduced to a thin disc since the light only needs to travel small distances in Yb:YAG to reach notable amplification1. On the other hand, the spectral gain of Yb in YAG is so small that these disk lasers can only emit picosecond pulses. When hosted in vanadate (YVO4) crystals, the emission spectrum gets much broader, making Yb:YVO4 the material of choice for femtosecond lasers in the 1030-nm region. Also, the gain of Yb:YVO4 is considerably smaller compared with Yb:YAG — hence long laser crystals are needed to reach enough amplification.
As a host, crystalline material is preferred because of its typically higher thermal conductivity and damage threshold compared to glass. Glass manufacturing, however, is much easier and therefore less expensive and available in large dimensions2. Besides the selection of an appropriate laser media mode locking, control of dispersion effects and amplification are important to build a powerful ultrafast laser.
Mode locking
Consider a Ti:sapphire crystal in a laser cavity that is pumped by a continuous wave (CW) laser. If the cavity is correctly aligned, the laser will start oscillating in the free running regime, which means it will oscillate simultaneously and continuously at all resonance frequencies of the cavity within the emission spectrum of the Ti:sapphire crystal. To move the laser from the CW regime to the pulsed regime, all the modes of different frequencies need to be locked on a fixed phase relation between each other (mode locking).
Mode locking can be done in two ways. In active mode locking, the resonator losses are modulated synchronously to the round-trip time of the resonator cavity, for example, by an acousto- or electro-optic modulator. In passive mode locking, resonator losses are modulated by a saturable absorber or a Kerr lens.
For a saturable absorber, the absorption losses decrease with increasing light intensity. Therefore, when the laser starts oscillating continuously, intensity spikes, which are found in the laser noise, have fewer losses than lower or average intensities. After many round-trips, a single pulse remains3. The pulse will oscillate in the cavity and as it passes the output coupling mirror, a small portion of the pulse will be emitted. Given a typical resonator length of 1.5 m, the round-trip length is 3 m. Thus the pulse, traveling at the speed of light, will pass the output coupler 100 million times a second, yielding a 100-MHz pulse repetition rate.
Dispersion
Ultrashort pulses take round-trips in the cavity, passing all optical elements within the cavity at least once (for a ring resonator) or twice (for a linear cavity) per round-trip. Since the pulse consists of many colors, it will experience group delay dispersion as typically red light travels faster than blue light through optical media. This will broaden and chirp the pulse, which needs to be compensated by either a pair of prisms or diffraction gratings, or by so-called chirped mirrors for which the penetration depth of red light is deeper than for blue light.
Amplification
Due to the high repetition rate of 80 to 100 MHz, peak intensities of ultrashort pulses emitted by an oscillator are rather small, even if the oscillator yields modest average power. Since many applications, especially in material processing, need much higher peak intensities, it is essential to amplify the ultrashort pulses. This amplification is realized by a master oscillator power amplifier (MOPA) system in which ultrashort pulses of a master oscillator are coupled into an amplifier. Laser media with broad spectral gain often exhibit low gain, so the pulse needs to travel a very long distance through the media to gain considerable amplification.
The most widespread amplifier type for femtosecond pulses is the regenerative amplifier. In this type of amplifier, a Pockels cell picks one pulse out of the 100-MHz pulse train emitted by the seed oscillator into the amplifying resonator where it takes many round-trips through an amplifier crystal. Both end mirrors of the regenerative amplifier are highly reflective to keep the losses per round-trip as low as possible. With each round-trip and each passage through the amplifier crystal, the pulse gains more energy. For Yb:YVO or Nd:Glass the pulse takes approximately 100 round-trips until it is coupled or dumped out again.
Ultrashort pulses can reach very high peak powers relatively quickly. To avoid damage to optical elements, pulses are stretched before they are coupled into the amplifier or stretched while they are inside the cavity by using the group delay dispersion described in the previous section. In the first case, a combination of gratings stretches the pulse. In the second, optical elements inside the amplifier cavity stretch the pulse, mostly the transparent elements like the Pockels cell or the amplifier crystal. The stretched pulses have the same energy as the ultrashort pulses but much lower peak intensity since they have a longer duration. The amplification of stretched pulses is often referred to as chirped pulse amplification. After the amplified stretched pulses are dumped from the amplifier cavity, they need to be compressed again, which is done most often with a pair of gratings4.
To avoid amplified pulses from running back into the seed laser and causing damage or instabilities, a Faraday isolator decouples the seed oscillator from the amplifier.
The key to fast switching
Particularly in laser material processing applications, ultrafast systems not only need high pulse peak powers but also they can benefit from high repetition rates that reduce the process time per work piece. The Pockels cell picks the pulses from a pulse train emitted by the seed laser and determines the repetition rate. Given a typical cavity length of 1.5 m and 100 round-trips, the pulse stays in the cavity for approximately one microsecond; theoretically, approximately 1 million pulses per second can be amplified. Historically, the piezo effect prevented these high switching rates. The Pockels cell consists of an electro-optic crystal that, by applying high voltage, rotates the polarization of one single pulse out of the 100-MHz pulse train. This single pulse is then coupled into the amplifier via a polarizer.
When high voltage is applied to the Pockels cell, the electro-optic crystal gets deformed and stressed due to the piezo effect. This stress will cause additional birefringence via the elasto-optic effect, which will disturb the originally desired electro-optic effect. Since the pulses emitted by the seed laser are only separated by approximately 10 ns, a very fast, precise switching Pockels cell is required, which means the piezo ringing should be as small as possible. So, electro-optic materials would be chosen in this case where piezo effect is pronounced very weakly, like beta barium borate (BBO) or rubidium titanyle phosphate (RTP).
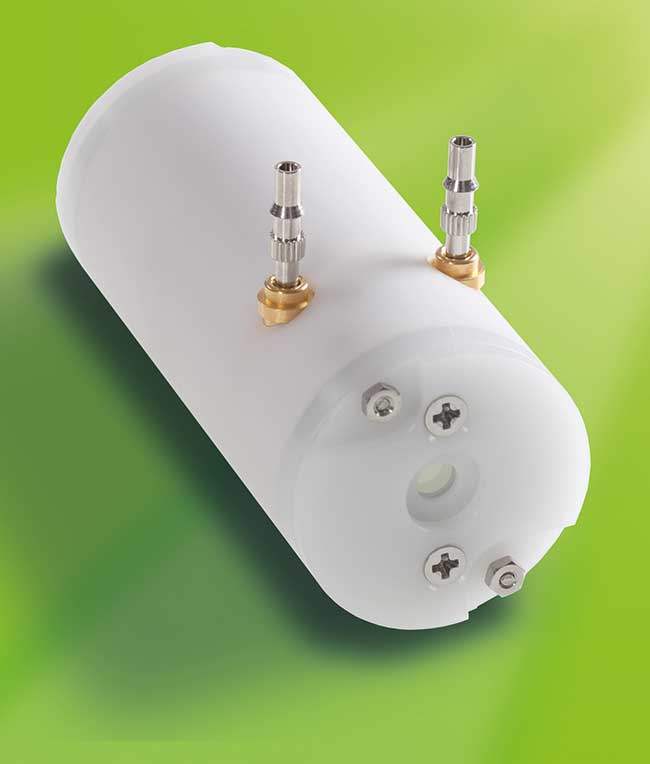
Figure 2. Qioptiq’s DBBPC HR Pockels Cell, with two Beta Barium
Borate (BBO) crystals for switching frequencies up to 1.3 MHz. Courtesy
of Qioptiq, an Excelitas Technologies Company.
The switching voltage of BBO is much higher than for RTP; however, it has an easy-to-compensate group delay dispersion and a superb absorption coefficient, making it the perfect choice for high-power laser systems. Up to approximately 300 kHz BBO can be used relatively easily as a very precise switching electro-optic crystal. At higher frequencies, though, even small piezo ringing effects will cause trouble with the ringing of the crystal lattice dissipating as heat. For example, at 750 kHz the crystal can get heated up to 130 °C. This heat will cause thermal expansion and twisting of the Pockels cell housing, which results in misalignment of the crystal or damaged Pockels cell. Water cooling may drain the heat, but since water and high voltage do not mix well, the design of a water-cooled cell is too complicated to be economical.
New BBO Pockels cells have recently been developed with improved thermal management and very stiff and temperature-stable housing (Figure 2). Together with BBO crystals that have the smallest possible absorption, this Pockels cell represents a new benchmark in electro-optics for fast switching in the MHz range and the resulting high-average laser powers.
The Faraday isolator
As mentioned before, the Faraday isolator is a key element in a MOPA system since it decouples the amplifier from the oscillator. In many regenerative amplifier configurations, a Faraday isolator is also used to couple the pulse into the amplifier and dump the pulse back out again (Figure 3).

Figure 3. Setup of a regenerative amplifier. A Pockels cell combined with a quarter-wave plate and a thin-film polarizer (TFP) act as an optical switch. The Faraday rotator is used for separating input and output pulses. Courtesy of R. Paschotta (2008). Encyclopedia of Laser Physics and Technology, p. 632. Berlin: Wiley-VCH Verlag.
Since laser powers have increased dramatically and UV conversion of the amplified short pulses has become more popular, laser manufacturers are placing greater importance on the low-outgassing properties of components within their systems. Typically, Faraday isolators are manufactured with many adhesives, which are among the materials with the worst outgassing behavior. Recently, adhesives have been avoided wherever possible and if they cannot be avoided, they have been replaced with vacuum-compatible adhesives, which resulted in a complete new series of low-outgassing Faraday isolators (Figure 4).

Figure 4. Qioptiq’s low-outgassing Faraday isolator helps to increase the live time of encapsulated, high power and UV short pulse laser systems. Courtesy of Qioptiq, an Excelitas Technologies Company.
For years, femtosecond pulses have been the shortest events that could be produced artificially by humans and hence, the laser systems have been operated at the edge of technical and physical feasibility. Today, technical issues are steadily being resolved and prices are reducing per mW output power, opening up exciting new frontiers in femtosecond technology for a wide variety of new applications.
References
1. W. Koechner (1976). Solid-State Laser Engineering, p. 87. Berlin: Springer Verlag.
2 W. Koechner (1976). Solid-State Laser Engineering, p. 57. Berlin: Springer Verlag.
3. R. Paschotta (2008). Encyclopedia of Laser Physics and Technology, p. 507. Berlin: Wiley-VCH Verlag.
4. C. Lupulescu (2004). Femtosekunden-Analyse und Rückkoppelungskontrolle von molekularen Prozessen in organometallischen und alkalischen Systemen, p. 22, Freie Universität Berlin dissertation.
Meet the author
Markus Fegelein earned his Diplom degree in physics from the Swiss Federal Institute of Technology in Zurich (ETHZ). During his 10-year tenure at Linos and later on Qioptiq, Fegelein has served as product manager for Faraday isolators, Pockels cells and electro-optic modulators; email: [email protected].