An IR imaging system employing a narrow spectral band brings hidden images to light, broadening IR imaging’s applications.
Austin Richards, FLIR Systems, Commercial Vision Systems
Spectral selection is a powerful tool that enhances conventional imaging tremendously. Most imaging systems, including the human eye, are designed to image light over a broad range of the spectrum. This makes the system more sensitive to the available light, but can often wash out details or information of interest.
By narrowing the color or spectral range, one can see things that are otherwise concealed or not apparent to a broadband imaging system. A simple example in the visible spectrum is the detection of bloodstains by crime scene personnel. Blood-stains can appear quite faint when imaged with a white light source such as a flashlight. Placing a blue filter over the flashlight makes the stains appear quite dark and easy to detect, since hemoglobin is highly absorbing in the blue portion of the spectrum.
Infrared imaging lends itself particularly well to spectral selection, because molecular materials exhibit very strong absorption and emission features in the infrared wavebands, making it possible to ascertain chemical composition of a material by imaging it at various wavelengths (Figure 1).

Figure 1. The graph indicates atmospheric transmission as a function of wavelength in the UV, visible and IR bands. Among the molecular absorbers displayed, water is the dominant one.
Infrared spectral selection applications also include imaging through opaque materials such as paint, or detecting materials that are transparent to the human eye, such as propane gas leaks from a tank or line.
Detector selection
Measured in nanometers or microns, the IR spectrum is a large portion of the electromagnetic spectrum, spanning two orders of magnitude in wavelength, from about 1 to 100 µm. The majority of IR imaging takes place between the spectral edge of human vision at 0.75 and 14 µm. Beyond 14 µm, special detectors cooled to very low temperatures are required, and the atmosphere becomes very opaque. Only a few space-based systems or special short-range imagers work in this portion of the spectrum.
The conventional IR spectrum begins with the near-infrared band, which ranges from 0.75 to 3 µm (there are variations in the definition of the near-IR range across different industries that use NIR technology). The midwave-IR, or MWIR band is the 3- to 5-µm range, and the longwave-IR, or LWIR band is 5 to 14 µm. Commonplace, low-cost imagers with silicon CCD and CMOS sensors can be used to image in the NIR band up to about 1.1 µm in wavelength. These silicon detectors usually have their peak sensitivity around 800 nm and also see visible light, which may need to be filtered out, depending on the application.
Beyond 1.1 µm, the silicon detector material becomes transparent as the corresponding photon energy drops below the bandgap energy of the semiconducting material. Operating beyond 1.1 µm with a silicon CCD or CMOS camera requires coating the sensor with a waveshifting phosphor, which greatly reduces quantum efficiency. This is not a problem in applications where there is an abundance of signal, as in beam profiling of 1.55-µm lasers for optical fiber communications, for example, but it is not very useful for imaging near-IR scenes.
A better alternative is indium gallium arsenide (InGaAs), which images in the 0.9- to 1.7-µm range, or near-infrared mercury-cadmium-telluride (HgCdTe) detectors, which can be designed to image from 0.8 to 2.5 µm. InGaAs can operate out to 1.7 µm and generally does not require cooling for most applications. Instead, the sensor temperature is stabilized with a thermoelectric cooler to a convenient temperature below ambient, such as 0 °C. The temperature stabilization at this lower temperature reduces dark current and makes the output signal stable as well. Cameras operating from the visible spectrum out to about 2.5 µm can use lenses made of conventional optical glass such as BK 7.
Indium antimonide (InSb) detectors operate in both the NIR and MWIR bands, between 1 and 5.5 µm. They have very high sensitivity, particularly at wavelengths between 2.5 and 5 µm. InSb’s range of sensitivity is so broad that InSb cameras almost always require spectral selection filters built into the cooled sensor housing, since it is often not advantageous to detect such a broad range of the spectrum. The filter itself must be cooled just like the sensor or it will contribute unwanted noise from its own MWIR emission.
In an application like long-range surveillance, InSb will typically be operated with a cold filter that restricts the sensor to seeing in the 3- to 5-µm range only. Otherwise, a broadband InSb detector will see reflected NIR sunlight, or sun glints, which are often regarded as undesirable clutter in a thermal image. Similar concerns exist for near-IR applications involving a reflected or transmitted light signal. In those applications, detection of thermal IR emission above 3 µm is not desirable.
Consequently, most InSb camera systems are designed to operate either as a NIR imager in a range from 1 to 3 µm, or as a thermal imager in the 3- to 5-µm range, but not both. Any IR camera operating beyond about 2.5 µm in wavelength requires special and costly optics made of germanium and silicon instead of conventional glass, which is highly absorbing in the thermal-infrared bands.
The quantum-well infrared photodetector (QWIP), another type of sensor, is narrow by nature, typically having a spectral range of about 1 µm. This range and its peak wavelength are dependent on the QWIP’s physical structure, which can be adjusted during the fabrication process. Thicker layers result in sensitivities at longer wavelengths. Standard quantum-well detectors used for thermal imaging have spectral responses between 8 and 9 µm in the LWIR range. The 8- to 9-µm band is a convenient window through the atmosphere because there is little absorption from atmospheric gases. QWIP sensors can also be made to work in other wavebands for special applications, including bands in the 5- to 7-µm range where water vapor is extremely absorbing.
There are many other detector types available, but the above types are the most common commercially available detectors that work on the photovoltaic principle in modern IR camera designs.
Selective filtering
How is imaging in a narrow region of the IR spectrum accomplished? The simplest way is to place a narrow bandpass filter in front of a broadband IR imager. This approach works fairly well in the near-infrared region of the spectrum, where lenses and filters do not self-emit. Narrowing down the bandpass of the imaging systems will reduce the signal reaching the detector, requiring a more sensitive camera or a brighter light source. Spectral selection in the thermal-infrared portion of the spectrum is more complex because, as stated earlier, a filter placed in front of the sensor will tend to self-emit infrared light that the detector sees as background noise. In the case of cooled detectors, it is often necessary to place any narrow bandpass filters inside the detector assembly so that the filter itself cools down to the temperature of the detector. In an indium antimonide camera, that temperature is 77 K, the boiling point of liquid nitrogen. Both the filter and sensor are encased in an evacuated radiation shield to reduce extraneous thermally emitted light from the interior of the camera body.
Another approach to spectral selection that works in a controlled environment such as a laboratory is to restrict the wavelength range of the illuminator, which works very well in most near-infrared applications where the scene must be actively illuminated. The light source can be a tungsten lamp with an adjustable filter wheel in front of it. The filters are then selected to pass various desired ranges of wavelengths. A better but much more expensive spectrally adjustable illuminator is a tunable laser, which can provide light with a very high degree of spectral purity. A particularly useful laser illuminator uses an optical parametric oscillator (OPO) to vary the wavelength of the light emitted.
Chemical imaging
Chemical imaging is a spectral selection technique that combines the power of imaging with the power of spectroscopy. Conventional infrared spectroscopy involves measuring the reflectance or transmission of a sample of molecular material as a function of wavelength. Infrared light couples very strongly with molecules, since the energy associated with molecular bonds is on the same energy scale as infrared photons. One can say that infrared light is the language of molecules.
Traditionally, a beam of IR light illuminates a surface and the magnitude of the reflected light is measured. This technique scans only a single spot on the surface, which makes scanning a large heterogeneous surface very slow. In contrast, chemical imaging rapidly provides two-dimensional maps of chemical concentrations within an image frame. In the simplest incarnation, a broadband IR imaging system is fitted with an adjustable narrowband filter. Adjustable filters come in a variety of types, including diffraction gratings, Fabry-Perot filters, and liquid crystal variable retarder and polarizer combinations called Lyot filters.
Images are taken of a static scene through the filter at a number of successive narrow spectral bands or channels. The scene is illuminated by a spectrally broad light source such as a tungsten lamp or blackbody source. The resulting stack of images obtained is known as an image cube, and can be processed into a composition map of the surface through principal component analysis. This technique is used by the pharmaceutical industry to map the spatial composition of pills to ensure a uniform distribution of active ingredient throughout the pill structure.

Figure 2. Three images showing subcutaneous water in a woman’s face.
A simple example of chemical imaging is shown in Figure 2. Three views of a woman’s face clearly show the presence of subcutaneous water. The first image was captured in a 20-nm wide channel at 1100 nm, where water is not particularly absorbing. The second was captured at 1400 nm with a 20-nm width, where liquid water is highly absorbing. The difference between each image’s digital intensities results in the third image, which shows only the skin with some shading in the eyes. The subtraction is achieved with image-processing software that acts on the raw 14-bit digital images. The third image shows an 8-bit gray-scale representation of the raw, 14-bit difference image.
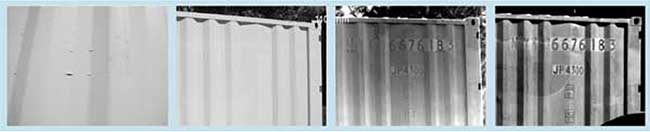
Figure 3. These four images show how infrared spectral selection can help users see through materials that are generally opaque to visible light, such as paint or thin paper.
Infrared spectral selection can also be used to see through materials that are generally opaque to visible light, such as paint or thin paper. The longer wavelengths scatter less in the material for a given thickness. Figure 3 shows four images of a shipping container. The two left images (one close-up, one distant) show it under visible light, where paint is opaque. The third image, captured in the 0.9- to 1.7-μm range with an InGaAs camera, shows the band where paint begins to become transparent. The fourth image (right), taken with an InSb camera with a cold filter operating in the 1.5- to 2.5- μm range, shows paint that is now quite transparent, allowing the numbers beneath to be read easily — a possible boon to port and border security specialists.