New platform and optics enable minimally invasive imaging deep within live animals.
Angela Goodacre, Olympus America Inc.
In vivo studies are the only way to accurately assess the interactions
of multiple cell types in the context of complex anatomical features. Cancer and
inflammatory disease, for example, represent the combined processes of cell migration
and recruitment, remodeling of extracellular matrices, flow and shear forces, and
complex modulation of multiple cytokines and chemokines.
New optics, coupled with the use of near-infrared
(650 to 900 nm) fluorescent probes, present exciting opportunities for in vivo imaging
of multiplexed fluorescent reporters in small-animal
models. This enables the simultaneous imaging of multiple cellular parameters and
the identification of mechanisms involved in complex biologic processes.
Scan head design
Confocal and multiphoton microscopy are used for
imaging within intact tissue, but even with the increased penetration of the infrared
wavelengths of multiphoton lasers, the scatter of photons emitted from fluorophores
limits the depth at which these technologies can achieve sufficient signal-to-noise
ratios.
Developed in collaboration with Ralph Weissleder and his colleagues at the Center for Molecular Imaging
Research at Massachusetts General Hospital in Charlestown, the Olympus IV100 intravital
laser-scanning microscope has a light path that is optimized for collecting light
from deep within living tissue.
High-resolution imaging over a wide range of wavelengths
also presents challenges. The optics used in the intravital microscope combine glass
and coating technologies that optimize the microscope for use from the blue through
the far-red wavelength range — and well into the near-infrared.
The advantages
of imaging in the near-infrared window are twofold: This region not only benefits
from the reduced scatter of longer wavelengths, but also avoids the wavelengths
confounded by tissue autofluorescence and by hemoglobin absorption. The intrinsic
fluorescence of biological molecules, such as those in blood vessels, can be useful,
however, in providing anatomic reference points.
The lasers used for the intravital
microscope are similar to those used in conventional laser scanning, with somewhat
higher intensity and an emphasis on the longer wavelengths. Based on the platform
of the Olympus FV1000 confocal laser-scanning microscope, the emission path of the
intravital system employs high-sensitivity photomultiplier tubes, along with ion-sputtered
emission beamsplitters and barrier filters for simultaneous acquisition of multiple
wavelength ranges. The small diameter of the optical fiber capturing emission from
the scan head, together with the relatively high numerical aperture of the objective
lenses, provides a degree of optical sectioning without a pinhole. Available for
the system are 10-mW, 488-nm argon-ion; 13-mW, 531-nm diode-pumped solid-state;
10-mW, 633-nm helium-neon; and 18-mW, 748-nm diode lasers.
Even when an animal is surgically opened,
the orientations and uneven surfaces of organs can cause difficulties when performing
conventional imaging with an upright microscope. Therefore, the system’s compact
scan head can be tilted for orthogonal imaging of internal organs. This enables
optimal imaging with minimal repositioning of the animal (Figure 1). With the instrument’s
enclosed system for optimal isoflurane anesthesia and temperature control, some
procedures are amenable to observation of up to several hours.

Figure 1. The scan head of an intravital laser-scanning microscope
tilts from 210° to 70° for optimal imaging of the internal organs of live
animal subjects.
Elias Gounaris and Rainer Kohler, also
of the Center for Molecular Imaging Research, have used the intravital laser-scanning
microscope to image protease activity along with neovascularization
to investigate tumor progression in a mouse prone to develop adenomatous polyps
and colon carcinoma. In this mouse model, adenoma formation was directly imaged
using fluorescent probes from VisEn Medical Inc. of Woburn, Mass.: one to detect
cathepsin B activity; the other, to delineate the microvasculature.
Following surgical opening of the abdomen, a loop
of gut was positioned for imaging using a 10x dry objective.
Images were acquired from a Z-stack over a 150-μm range (Figure 2). In some
studies, two excitation channels were used; in others, a third channel was acquired
simultaneously to provide anatomic morphology.1
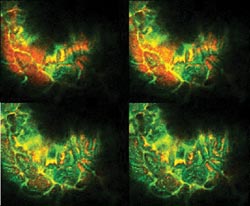
Figure 2. These representative images are from a Z-stack imaged through
the gut wall using the intravital microscope and a specialized 10x objective lens.
Courtesy of Elias Gounaris and Rainer Kohler, Massachusetts General Hospital Center
for Molecular Imaging Research.
The use of long-wavelength excitation (633 and
748 nm), along with the long-wavelength emission of the dyes, enabled excellent
image contrast and resolution, even with direct imaging through the intact wall
of the gut. The system allowed the researchers to conduct their studies with the organs in situ rather than having to work with exteriorized organs.
Specialized objectives
For minimally invasive imaging, the intravital
microscope’s platform can be coupled with Olympus’ MicroProbe objective
lens, which provides a sharp contrast to conventional high-resolution objective
lenses.
The large barrels of conventional lenses
require extensive surgery to expose enough of the subject animal’s internal
organs, and many such studies must be performed with the organs completely exteriorized.
Exposing an animal to this level of surgical trauma reduces the possibility of longitudinal
studies in a single animal and can affect the biological relevance of data, especially
in investigations of the inflammatory response. The ability to follow disease progression
or response to treatment in a single animal is attractive not only because of the
potential to intervene, but also because of the smaller number of animals required
for preclinical studies.
The slim barrels of the MicroProbe lenses allow
close apposition of the front lens element to the tissue without fear of affecting
the organ or causing undue trauma to the animal. The lenses have an outer diameter
of 1.3 mm and extend up to 2 cm in length, permitting insertion through a tiny incision
into the body cavity for imaging organs in situ. Importantly, longitudinal studies
often can be enabled by the use of minimally invasive techniques such as keyhole
surgery and subsequent suture.
The water-immersible objectives can
yield Z-stacks that extend 200 μm into an intact organ. There are three lenses
in the series (Figure 3), all designed to satisfy in vivo imaging applications:
a 27x, 0.7-NA, 3.5-mm-diameter lens with a 220-μm field of view; a 20x, 0.5-NA,
1.3-mm-diameter lens with a 200-μm field of view; and a 6x, 0.14-NA, 1.3-mm-diameter
lens with a 670-μm field of view.
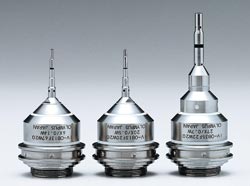
Figure 3. MicroProbe objectives have especially thin barrels, facilitating in vivo imaging
deep within tissue.
Each of these lenses can be sealed
with an O-ring cap for immersion in sterilization fluid,
using a dedicated protective frame. The spring loading is contained within the scan
head. Each objective adheres to Royal Microscopical Society thread standards and
is transmissive and corrected for a wide range of wavelengths. The transmission
at 1000 nm is at least 80 percent as great as transmission at 500 nm.
Vascular leakage is an indicator of tissue damage
due to ischemia. The increased permeability of small vessels occurs because of intercellular
gaps and necrosis of endothelial cells lining the vessel. Research into these areas
can help determine the processes underlying this inflammatory response, which often
results in life-threatening events. In a mouse model of induced intestinal reperfusion
injury, Herlen Alencar and Umar Mahmood of the imaging research center used the
intravital laser scanning microscope to image leakage from microvessels in the villi
of the jejunum.2
After surgically opening the abdominal
wall, they inserted the objective through a small incision in the wall of the jejunum,
into the lumen and directly against — but not penetrating — the mucosal
surface.
Comparing experimental, control and
sham-treated mice, the investigators examined the role of the inflammatory response
and the possibility of mitigating it in cases of reperfusion injury. In experimental
mice, significant leakage of the vascular probe was observed as early as 20 minutes
after reperfusion. They then used the imaging system to view the effects of an experimental
treatment to prevent damage resulting from reperfusion injury (Figure 4).
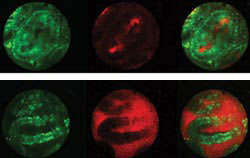
Figure 4. Leakage of a fluorescent-labeled vascular probe from microvessels
within the villi of the jejunum was imaged using intravital laser-scanning microscopy.
To image circulation within the villi, a 1.3-mm-diameter objective lens with a 2.5-cm-long
barrel was inserted into a small incision in the wall of the jejunum and placed
in direct contact with the mucosal surface. The blood vessels within the lamina
propria of microvilli are identified by the circulating vascular probe (red), and
the enterocytes are stained by rhodamine 6G (green). Both channels are acquired
simultaneously but also are displayed separately for clarity. In the treated animal
(top), the vascular probe is retained within vessels; in the untreated control (bottom),
there is leakage into the lumen. Courtesy of Herlen Alencar, Massachusetts General
Hospital Center for Molecular Imaging Research.
Imaging with the intravital microscope
showed that the agent is retained in the blood vessels inside the colonic villus.
In contrast, unmitigated leakage was observed in the saline-treated control. By
quantitatively imaging vascular leakage in this way, researchers can better assess
compounds selected for preclinical trials.
When looking at complex interactions
of cells with regard to tissue architecture, imaging in vivo and in situ is beneficial.
Cancer and inflammatory disease are significant human health problems, and their
pathophysiology is one such area of research. In vivo imaging has the potential
to elucidate the underlying mechanisms of disease and to offer direct observation
of the effects of therapeutic intervention.
Only recently, with the development
of new in vivo imaging systems, has such minimally invasive surgery imaging been
possible with minimal trauma to the animal. The acceptance of these methodologies
will enable better imaging of reporters of molecular activity, which, in turn, can
be immensely valuable in target and pathway validation.
References
1. M. Zhang et al (Jan. 3, 2006). Identification
of the target self-antigens in reperfusion injury. J EXP MED, pp. 141-152.
2. H. Alencar et al (November 2005).
Novel multiwavelength microscopic scanner for mouse imaging. NEOPLASIA, pp.
977-983.
Meet the author
Angela Goodacre is group manager for applications
systems, Bio Business Development Div., Life Science Group, Olympus America Inc.,
Center Valley, Pa.; e-mail: [email protected].