Next-generation high-speed IR readouts and new detector materials give IR cameras the needed speed and dynamic range for high-speed thermal testing on the range.
CHRIS BAINTER, FLIR SYSTEMS
For many years, high-speed visible cameras offered the ability to stop motion on fast-moving objects and provided unique insight through slow-motion playback for high-speed applications such as ballistics, munitions and tracking applications. Unfortunately, their thermal IR camera counterparts didn’t offer the snapshot speeds, high frame rates, and wide temperature measurement ranges necessary to be useful for these same testing applications. But with next-generation high-speed infrared readout integrating circuits (ROICs) and new strain layer superlattice (SLS) detector materials, there are finally commercial off-the-shelf (COTS) infrared cameras that can provide the much-needed speed and dynamic range to meet the challenges of high-speed thermal testing applications on the range. The following will discuss the benefits of these next-generation ROIC and detector material technologies and how they are pushing the boundaries on high-speed thermal testing capabilities.

Thermal image of munitions testing.
Legacy thermal cameras: slow frame rates
There are two key challenges to recording high-speed events with legacy thermal cameras. The first is that ROICs don’t offer short-enough snapshot speeds to stop motion on high-speed objects, leading to blurring in the imagery and subsequently inaccurate temperature measurements. The second is that older ROICs simply don’t have fast enough frame rates. If sampling isn’t done fast enough, aliasing in the data can occur and critical temperature spikes could be missed during tests.

High-speed thermal camera image of .45 bullet hitting a steel plate.
Over just the past two years, there have been significant gains in ROIC design and detector material, leading to significant breakthroughs in infrared camera technologies for high-speed thermal applications. New ROIC designs now allow for integration times below 270 ns — sufficient to stop motion on many high-speed range applications, such as ballistics testing on a .223 round from an AR-15, impact and scoring applications, munitions testing on high explosives or muzzle flash measurements. The new design also allows for frame rates of thousands — or even tens of thousands — of frames per second, depending on the number of pixels per frame selected by the user. At those speeds, IR cameras are still only working at about 1/10th of the speed of that of a visible camera; even so, this is a giant leap forward and makes IR cameras now useful for high-speed range and lab testing applications.
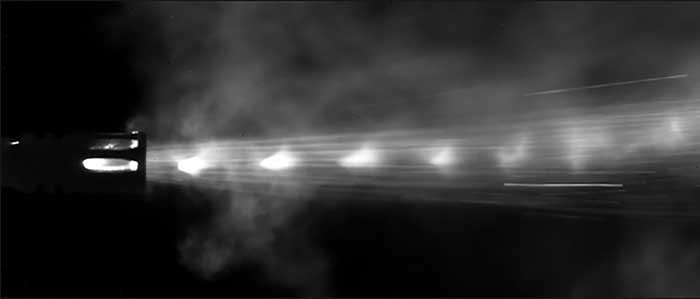
Mach diamonds exiting a barrel.
Along with faster frame rates and higher speeds, newer ROICs are more linear across their entire dynamic range. This means that for a given increase in radiant energy, there’s a corresponding linear increase in count values for the camera. Older ROICs have a roll-off at the low end and high end of the dynamic range. The response looks like an “S” curve. This is critical because, for many high-speed targets, the detector needs to be set at a short integration time to stop motion and allow for fast frame rates, but if the target is not hot enough, it won’t supply enough photons for the detector to push into the mid well-fill range. These lower-temperature targets require operation at the very bottom of the well-fill. The older-generation ROICs, being nonlinear at low well-fill, meant the nonuniformity and calibrations would not calculate correctly and measurements on that data were nearly useless. Newer ROICs are linear all the way to near zero well-fill. This remedies the problem and allows for crisp imagery and accurate temperature measurements in this shorter well-fill range.
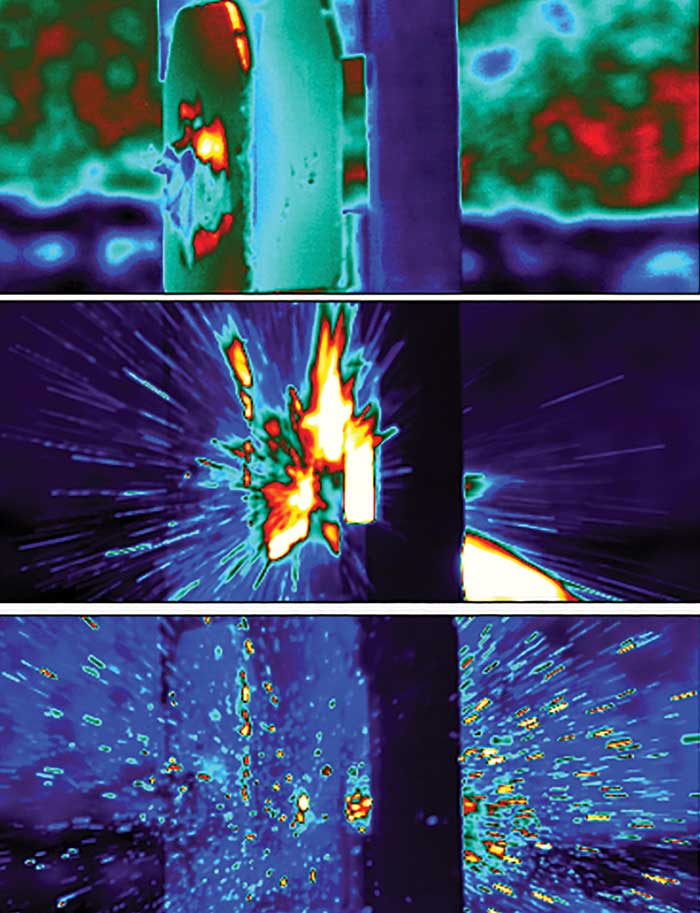
One of the advantages of thermal over high-speed visible imaging is that shrapnel is much easier to see due to the high thermal contrast against the background. Here, an AR15 55-grain, .233-caliber round penetrates plated body armor.
Long-wave SLS detectors
In recent years, there’ve been significant readout and camera electronic advances that push the limits of resolution, speed and sensitivity; however, it wasn’t until recently, with the introduction of type II SLS, that the industry saw a new detector material enter the market that offers equally significant advances in overall thermal camera performance in line with their ROIC and camera electronic counterparts. With the integration of SLS into commercially available thermal camera solutions, a new longwave-IR (LWIR) solution offers significant improvements in speed, temperature range, uniformity and stability over current detector material incumbents.
While SLS works in both the LWIR and midwave-IR (MWIR) bands, the biggest performance benefits come when SLS is filtered to the LWIR bands exclusively. In fact, one of the key benefits of SLS is its short integration times, or snapshot speeds, over other infrared camera materials. In comparison to similar MWIR indium antimonide (InSb) detectors, SLS has 12.6× faster snapshot speeds than InSb detectors when imaging the same temperature range.
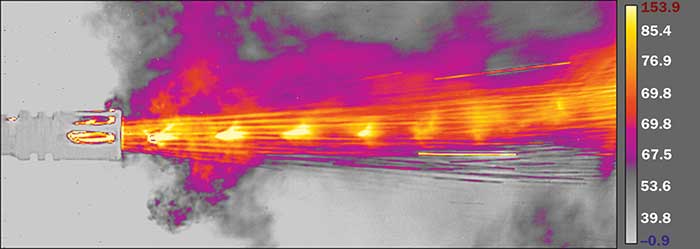
Thermal Image of AR-15 muzzle exhaust.
As discussed previously, sometimes there is a scene that is changing fast, but is not sufficiently hot to achieve the well-fill needed for short snapshot speeds and high frame rates. For example, if a MWIR camera images at 640 × 512 at 1000 frames per second but requires an integration time of 1.2 ms due to the bandpass in which the camera operates, that would prevent achieving the camera’s full frame rate potential. In comparison, an LWIR SLS detector will get more photons in the LWIR, which allow for shorter snapshot speeds and faster frame rates.
A second benefit of LWIR SLS thermal cameras is their wider temperature bands. The LWIR SLS camera has a starting temperature range from −20 °C to 150 °C with one integration time (Table 1). To achieve the same temperature band with MWIR InSb, you’d need to cycle through (superframe) three integration times, each representing a different temperature range. Having to cycle through three temperature ranges means three times more work when calibrating the camera as well as one-third slower overall frame rate. Cycling through three temperature ranges to superframe them into one complete −20 °C to 150 °C temperature range results in one superframe image per three frames captured from the camera.

One can also measure higher temperature ranges before needing a neutral density (ND) filter with LWIR SLS thermal cameras. The SLS camera evaluated allowed for measurements up to 650 °C before requiring an ND filter, whereas an MWIR InSb camera only measures up to 350 °C before it requires an ND filter.
This is partly a function of the SLS operating in the LWIR band vs. the InSb operating in the MWIR. Notice the spectral emissive power of a 30 °C ideal blackbody (Figure 1). The area under the curve represents the power within that waveband, which shows a much larger area for the LWIR than the MWIR. As objects heat up, this curve’s peak shifts to the left and tails off to the right. The change in power in the LWIR band is less dramatic over a range of temperatures than the more dramatic change that happens in the MWIR band. This is how the SLS LWIR is able to avoid over- or underexposure for a given integration time in comparison to the MWIR InSb detector. In comparison, the change in power in the MWIR band is substantial and saturation soon occurs for a single integration time. The SLS allows users to tackle challenging applications where the target heats up across a wide temperature range quickly, such as a combustion research application.

Figure 1. Spectral emissive power of a 30 °C ideal blackbody.
However, operating in the LWIR is not the only factor. LWIR mercury cadmium telluride (MCT) detectors, for instance, also are limited in their ranges, similar to MWIR InSb detectors. Notice that the LWIR MCT cameras also have both shorter individual ranges per integration time as well as limitations on how high they can measure before needing an ND filter to cut down the signal.
When looking at high-speed range-testing applications, one of the biggest advances offered by LWIR SLS cameras in comparison to incumbent LWIR-cooled detector options is the dramatically improved uniformity and stability through cooldowns. A main drawback to LWIR MCT cameras is the poor uniformity and stability. When the user turns on an LWIR MCT camera, the last uniformity correction performed needs updating (Figures 1 and 2).

Figure 2. Mercury Cadmuim Telluride (MCT) image at start up.
This presents problems for field-based test-range applications that are simply not conducive to updating gain, offset and bad pixel maps due to environmental conditions; for example, if the camera is in a test chamber, in a blast zone or another location that’s not easily accessible. LWIR SLS, on the other hand, operates much like MWIR InSb: Simply turn it on and start testing. The uniformity correction done previously in the lab works just as well in the field with no extra image uniformity updates, beyond possibly a one-point-offset update using the internal nonuniformity correction (NUC) flag inside the camera. The NUC also holds well through multiple cool-downs over a long duration. The camera tested for this article has not required a new NUC since initial fielding of the camera more than a year ago.

Figure 3. Strain layer superlattice (SLS) image at start up.
Shorter integration times, faster frame rates
The advances in ROIC design allowing for shorter integration times, faster frame rates and better linearity at low well-fill, coupled with the advances in new detector materials such as LWIR SLS, high-speed thermal cameras, are finally becoming the standard for high-speed thermal
measurement on the government test range. The SLS detectors fill a niche in the performance spectrum by offering shorter integration times, wider temperature bands and better uniformity than MWIR InSb or LWIR MCT materials.
Meet the author
Chris Bainter is the global business development director for Flir Systems, a world leader in the design, manufacture and marketing of sensor systems. Chris has a bachelor’s degree in computer engineering from Kansas State University and an MBA from the University of Southern California; email: [email protected].