Bill Fester, Olympus America Inc.
Applications from cell dynamics and single-molecule events to vesicle and protein tracking are all within the realm of total internal reflection fluorescence (TIRF). The technique is so named because it involves total internal reflection of the excitation light.
The method requires no X-, Y- or Z-stage motorization because the cells are stationary. The evanescent wave in the illumination extends only about 100 nm from the coverslip/ water interface, so any area of interest must be within that region. That means growing cells on a coverslip. If one simply places a coverslip on a cell to seal it to the slide, there is no guarantee that the cell — or most of one side of the cell — will be that close to the surface.
Total internal reflection fluorescence microscopy is being used in some applications to study movement of the cell or cellular components. Cell adhesion and the dynamics of actin filaments are two that may require X-Y motorization.
Reflection in this type of microscopy occurs when the light hits the discontinuity in refractive indices. The refractive index of the front lens of the objective, of the immersion oil and of the glass in the coverslip is about the same (~1.515). Light passes through all without bending, but faces a strong gradient once it reaches water (refractive index of ~1.33) and/or cellular material (~1.38) on the other side.
Total internal reflection occurs when light approaches this junction at an angle greater than the critical angle, and this establishes an evanescent wave on the far side of the junction. Using a laser, the wave has enough energy to excite fluorochromes on the other side of the junction to within about 100 nm.
This zone results in a selective optical section. Often in optical sectioning, the goal is to isolate multiple planes of focus in Z, but this case involves just one plane because the wave penetrates only from the junction. However, it is a very good, very isolated plane. No light travels beyond this plane to excite other fluorochromes in the cell, so the result is all signal with little noise.
Objectives
Even with these limits, the technique still offers imaging flexibility. The first objective developed for the process has a numerical aperture of 1.65. Olympus Optical Co. Ltd. of Tokyo first constructed this lens as a custom order for Dr. Susumu Terakawa at Hamamatsu University School of Medicine in Japan in the mid-1990s. He discovered that it worked well for total internal reflection fluorescence microscopy. Olympus America Inc. in Melville, N.Y., introduced the product to the US market in late 1998, and Dan Axelrod, professor of physics at the University of Michigan in Ann Arbor, used it to develop the technique.
The 1.65-NA objective requires a special high-refractive-index glass and immersion oil, both of n = 1.78. The oil is rather uncommon and slightly more potent than standard immersion oil, and the glass is custom-made in Japan and expensive when cut into coverslips. These two factors led Olympus to develop a 1.45-NA objective.
To achieve the conditions for total internal reflection fluorescence microscopy with live cells (i.e., the cell is in water), it was necessary to illuminate beyond the 1.38 NA in the back focal plane of the objective (see figure). And yet, while theoretically possible to use a traditional 1.4-NA objective, this turned out to be impractical.
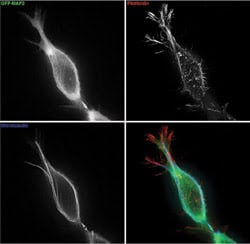
Kenneth Fish of the Scripps Research Institute in La Jolla, Calif., imaged this primary hippocampal neuron. The microscopy setup included an argon/krypton laser and an Olympus total internal-reflection fluorescence objective (1003, 1.65 NA). The only image processing was the removal of an inherent interference pattern using a standard algorithm.
With a 1.45 NA, though, there is enough room in the back focal plane to accomplish total internal reflection microscopy illumination and still maintain standard immersion oil and standard coverslip glass. This is a great benefit to labs doing high-volume work, especially multiuser facilities, which is perhaps why this lens accounts for about 70 percent of sales.
The 1.65-NA objective still has its place because the larger refractive index difference between the cover glass and specimen results in decreased depth of the evanescent-wave penetration (which is beneficial for optical sectioning). The high numerical aperture also captures more emission light for brighterimages.
Finding flexibility
One recent trend is toward using multiple fluorophores as they are used in traditional fluorescence microscopy. When a researcher wants to track two or more events on a cell membrane, the molecules, proteins, etc., are labeled with different fluorochromes so they can be distinguished by color. Illuminating one or more fluorochromes at a time requires multiple lasers and a switching system to select one or the other or both at any given time. This allows collocalization studies or simple tracking.
Olympus now offers a laser combiner that allows three lasers to be on simultaneously, and a system of filters and shutters enables one, two or all three to come through the system. Shuttering the lasers turns illumination on and off.
This is where motorization is handy. Users would rather have the computer open and close a shutter, especially if a time series is being captured. For example, when imaging a time series of two colors with a somewhat fast sampling rate — say, 50 cycles in 30 seconds — each cycle requires two images. So the computer can turn on one laser, trigger the camera, close the shutter, open the other, trigger the camera again, close the shutter, open the other and so on. This is not practical manually.
Users also might want to switch between wide-field and total internal reflection fluorescence microscopy, and capture those images rapidly. Although this is not yet a commercial option, researchers are developing new techniques all the time. The wide-field image could serve as a reference to something going on in the cell body, which could provide a road map for the activity indicated by the total internal reflection image if the two are superimposed.
Olympus is working to give researchers motorization like this in all areas of microscopy. On the imaging side, there may be no call for motorization with this technique because the specimen is generally not moving, and because there is only one plane in Z (no focus drive) and only one objective.
Contact: Bill Fester, director of biomedical marketing, Scientific Equipment Group, Olympus America Inc., Melville, N.Y.; +1 (631) 844-5041; fax: +1 (631) 844-5111; e-mail: [email protected].