The optical spectroscopic technique uses laser illumination to identify the infiltrative edges of glioblastoma tumors in the brain, helping to inform resection surgery.
SILVIA NOBLE ANBUNESAN, ALBA ALFONSO-GARCIA, AND LAURA MARCU, UNIVERSITY OF CALIFORNIA, DAVIS
Fluorescence lifetime imaging (FLIm) is an optical spectroscopic technique that is well suited to interrogating biological tissues in vivo. By measuring the time-resolved fluorescence properties intrinsic to tissues, FLIm can distinguish between areas of different metabolic activity and structural composition. This becomes particularly significant when plotting intervention for cancers such as glioblastoma, the most aggressive form of brain cancer. Despite its very poor prognosis, maximum possible tumor resection — which is potentially enabled by FLIm — is responsible for prolonged survival rates. However, glioblastoma tumors do not have a well-defined border. Instead, they are characterized by a highly infiltrative edge into the surrounding eloquent tissue that harbors a mix of functional brain tissue with tumor cells and other cancer-associated molecular markers. This biochemical information can be hard to distinguish using other imaging modalities.
Figure 1. A fluorescence lifetime imaging system in the operating room. Courtesy of University of California, Davis.
There is a pressing need for clinicians to identify the infiltrative edges of glioblastoma tumors in vivo during tumor resection surgery to minimize the chances of recurrence and to improve the patient’s prognosis. Using a unique FLIm device developed in the Marcu Laboratory at the University of California, Davis, the autofluorescence properties of distinct brain tissue types were studied in patients. The inherent advantage of this label-free technique is its ability to identify areas of healthy brain cortex and white matter, tumor masses, and the infiltrative edges of glioblastoma tumors and other forms of brain cancer.
Fluorescence lifetime imaging is a simple, yet powerful technique. Upon laser illumination of a specific wavelength, certain tissue components emit fluorescence that can be measured. The laser illumination excites fluorescence from endogenous fluorophores present in biological tissue. The temporal and spectral properties of the emitted fluorescence are unique to the biomolecular and structural composition of biological tissue. More specifically, the lifetimes are associated with the local microenvironment of naturally occurring fluorophores.
The most prominent naturally emitting fluorophores that are detectable by this method are the metabolic cofactors nicotinamide adenine (phosphate) dinucleotide (NAD(P)H) and flavin adenine dinucleotide (FAD), as well as crosslinks (molecular bonds) in structural proteins such as collagen and elastin. By observing these fluorescence intensity and lifetime properties, relevant biological information can be inferred, such as molecular makeup and metabolic activity of biological tissue. This is especially useful in studying cancers, because cancerous tissue and cancer-associated injury (e.g., radiation-induced necrosis), dramatically alter tissue metabolic activity.
Shedding light on glioblastoma
Not only is glioblastoma the most aggressive type of brain cancer, it is also resistant to traditional radiation treatment and chemotherapy. It is classified by the World Health Organization as a Grade IV tumor, meaning cells are actively dividing. The survival rate is devastatingly low — only 6.8%. The unique feature of this disease that makes it so lethal is its highly infiltrative nature. Up to 90% of recurrences in glioblastoma patients occur within 2 to 3 cm of the resection margin1.
Figure 2. A diagram of the FLIm experimental workflow. Courtesy of University of California, Davis.
Despite the development of and experimentation with various cancer treatment methods, surgical removal is still the most common therapy for glioblastoma patients. Patient survival is directly related to the extent of resection. Extremely aggressive resection poses the risk of causing neurological damage, whereas inadequate resection (when cancerous cells remain) leads to the potential for tumor recurrence. Neurosurgeons are thus faced with a unique challenge in the operating room: to strike a fine balance between aggressive surgical resection and preserving tissues and systems that are needed in neurological activity. Therefore, technology that can accurately characterize the tumor infiltrative edge will directly affect a patient’s prognosis.
Fluorescence lifetime imaging can be integrated with the surgical workflow and allows for in vivo imaging of the tissue of interest in real time. The clinically compatible FLIm device developed in the Marcu Lab is based on a pulse sampling principle that is compatible with standard room light illumination and allows for fast acquisition of endogenous (weak) signals. Tissue excitation using short, intense (~0.1 to 10 μJ) pulses results in the generation of a large amount of fluorescence photons, which are detected by a high-bandwidth photodetector. This is followed by a fast digitizer that measures the resulting electrical transient signal in the order of picoseconds. Thus, full fluorescence intensity decays are recorded within a few microseconds2.
This approach is especially relevant in clinical settings, as the operating room illumination has a negligible effect on the acquisition of the signals. Therefore, the approach enables imaging and surgical work (resection) to happen simultaneously. Packaged into a portable and flexible instrument, this FLIm system has been used in the operating room for research studies (Figure 1). The system is flexible because various sterilizable fiber optic probes specifically designed for different surgical strategies — depending on the type of tissue (e.g., brain, head and neck, intravascular, or breast) — can be made compatible with the system. This enables the scanning of different tissue regions both in vivo and ex vivo. The design of hand-held fiber optic probes enables free scanning of tissue masses and cavities, producing results not achievable with bulky, stationary instruments. This is advantageous over wide-field or laser scanning systems, which lose features hidden from the field of view while capturing a wide-field picture of the region of interest. Such lost features may include crevasses in exposed brain cavities and restricted internal organs, such as vasculature and oropharynges that may be relevant for the operation.
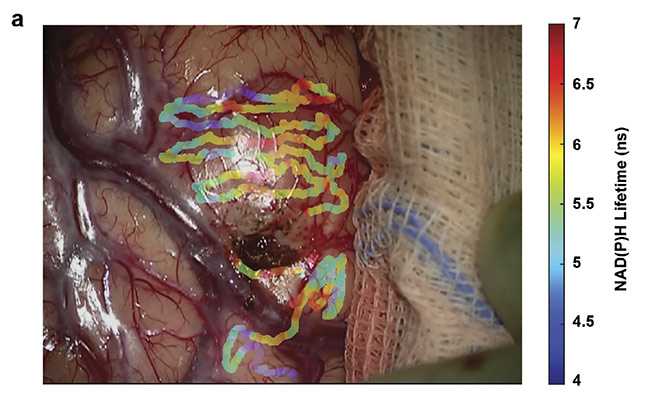
Figure 3. Overlays of nicotinamide adenine dinucleotide (NAD(P)H) lifetimes on a tumor-infiltrated cortical surface (a) and a visibly clear cortex (b) of a glioblastoma patient. Courtesy of University of California, Davis.
The FLIm system allows direct in vivo examination of a patient’s biological tissues during surgery, specifically for interrogating brain cancers. The instrument has safety settings in place to protect tissue from thermal damage, such that exposure to laser excitations is well within the maximum permissible exposure limit. Real-time data analysis is made possible by overlaying complex data that is processed by pretested and preloaded classification algorithms onto the surgical field of view. Figure 2 illustrates the workflow of the implementation of fluorescence lifetime imaging in the operating room, along with the subsequent data collection, analysis, visualization, and validation with ground truth provided by clinical pathologists.
FLIm vs. other methods
Current tools that neurosurgeons rely on to identify the infiltrative edge include preoperative radiological imaging — for example, magnetic resonance imaging (MRI) — and the surgeon’s experienced eye. MRI is a great medical imaging tool in radiology that can form pictures of anatomical and functional processes of the human body with high accuracy. But it does not provide the required sensitivity when it comes to detecting infiltrative edges in glioblastoma during surgery. Also, preoperative MRI does not account for brain shifts during craniotomy and provides no biochemical information to see the edge in real time. Although technological strides have been made in intraoperative MRI (iMRI), which makes it possible to locate abnormalities in tissues caused by brain shift during the craniotomy procedure, the technology is quite expensive. And the integration of iMRI imaging with the surgical workflow is complex and may interfere with patient care, due to required preparation protocols.
Fluorescence lifetime imaging can overcome these issues, however. It provides mesoscopic sensitivity to detect tumor infiltration, and the hand-held probe makes it possible to take point measurements of the entire tissue of interest in real time. The system can thus be seamlessly integrated within the surgical workflow because it is robust (it works under standard room light illumination) and does not disrupt other instruments. Combining FLIm with MRI in the operating room can provide powerful surgical guidance for the neurosurgeon to intraoperatively identify and remove maximum possible infiltration.
Building on current techniques
Currently a well-documented and FDA-approved fluorescence-based imaging technique for visualizing glioblastoma during surgery is the exogenous use of 5-Aminolevulinic acid (5-ALA) to induce visible fluorescence in tumor cells and provide contrast to detect the bulk of the tumor. A metabolic precursor, 5-ALA converts to protoporphyrin IX (PpIX), which has a bright pink fluorescence under violet-blue light. This intraoperative visualization of the tumor has enabled neurosurgeons to perform better resection, but it has limitations in the infiltrative zone that could be addressed by using FLIm. PpIX has a characteristic fluorescence lifetime that can be leveraged to detect and quantify lower signal contributions using FLIm.
Recently, a study on freshly excised brain tissue demonstrated that combining fluorescence lifetime imaging of PpIX and NADH (nicotinamide adenine dinucleotide) results in higher sensitivity to tumor detection than intensity-based 5-ALA visualization alone3. Measuring the time-resolved fluorescence of PpIX and the endogenous fluorophores NAD(P)H and FAD can help to better characterize high-grade and potentially low-grade tumors, especially in the infiltrative zones with low tumor cellularity.
Once contrast between the tumor and adjacent viable tissues has been established, the next step is to use fluorescence lifetime imaging to elucidate the relationship between the optical parameters observed and the metabolic state of the tissue under scrutiny. NAD(P)H and FAD are the predominant endogenous fluorophores in the brain. Alterations in energy metabolism in cancer cells lead to changes in the NAD(P)H and FAD fluorescence properties, but several other cancer-associated metabolic and genetic component changes may also affect the fluorescence signature of the tissue.
For example, isocitrate dehydrogenase (IDH) is a key enzyme in cellular metabolism. Mutations in IDH are common in certain brain tumors and have been identified as a factor leading to a favorable prognosis. Patients diagnosed with gliomas, including glioblastoma, who express IDH mutations have better prognosis post-resection than those without the mutation and respond better to chemotherapy. FLIm has the potential to clarify this distinction during surgery, which could immediately inform surgical resection strategy and clinical treatment, without the need to wait for histopathological molecular analysis.
Plotting a way forward
In conjunction with clinical fluorescence lifetime imaging research, there is a growing need for further understanding of the meaning of lifetime and spectral changes in the tissue in various types of brain tumors. Researchers expect to expand their database of glioblastoma cases and evaluate other low- and high-grade brain tumors and metastasis. As the complexity of the data increases, data modeling and machine learning techniques using artificial intelligence will lead to real-time processing and visualization of FLIm data that can be combined to provide a tumor probability map on the surgical field of view. This map will be usable in conjunction with other technologies. The authors have already successfully classified tumor tissue in head and neck cancer and are now working on an algorithm to aid in the study of brain tumors.
The goal of intraoperative fluorescence lifetime imaging is to provide clinicians with visual feedback of the biochemical data augmented on the reflective images of the tissue structure (Figure 3). In parallel, a conclusive understanding of the correlation between the biochemical makeup of tumor tissue along with the surrounding healthy tissue in the brain and the optical data obtained from the FLIm system needs to be attained. Current studies are aimed at expanding the database and analyzing data from various glioma diagnoses, tissue types, and grades of cellularity within the brain. Eventually FLIm could serve as an intraoperative adjunct tool that will provide information on the biochemical and metabolic activity associated with tumor-infiltrated regions to the neurosurgeons to achieve optimal resection outcomes.
Meet the authors
Silvia Noble Anbunesan is a graduate student at the University of California, Davis, where she obtained a master’s degree in electrical and computer engineering. She is pursuing her doctorate degree in biomedical engineering, and her current research focuses on clinical application of fluorescence lifetime imaging in studying brain cancer and metabolism; email: [email protected].
Alba Alfonso-Garcia, Ph.D., is an assistant project scientist at the University of California, Davis. She obtained her doctorate from the University of California, Irvine, working in the fields of multiphoton microscopy and coherent Raman microscopy. Her current research focuses on the clinical translation of fluorescence lifetime imaging for surgical applications; email: [email protected].
Laura Marcu, Ph.D., is a professor of biomedical engineering and neurological surgery at the University of California, Davis. She is also a fellow of numerous professional societies, including the Biomedical Engineering Society, SPIE, Optica (formerly OSA), and the National Academy of Inventors. Marcu’s group is best known for researching and engineering fluorescence lifetime-based instrumentation and multimodal imaging systems that enable studies of the molecular, metabolic, and morphologic changes in living systems, ranging from biological cells and animal models to human patients; email: [email protected].
References
1. A. D’Alessio et al. (2019). Pathological and molecular features of glioblastoma and its peritumoral tissue. Cancers (Basel), Vol. 11, No. 4, p. 469.
2. A. Alfonso-Garcia et al. (2021). Mesoscopic fluorescence lifetime imaging: fundamental
principles, clinical applications and future directions, J Biophotonics, Vol. 14, No. 6.
3. M.T. Erkkilä et al. (2020). Macroscopic fluorescence-lifetime imaging of NADH and protoporphyrin IX improves the detection and grading of 5-aminolevulinic acid-stained brain tumors. Sci Rep UK, Vol. 10, No. 1, p. 20492.