Surface-plasmon-coupled emission shows promise to expand its chemical and biological sensing applications.
Dr. Chris D. Geddes, Dr. Ignacy Gryczynski, Dr. Joanna Malicka, Dr. Zygmunt Gryczynski and Dr. Joseph R. Lakowicz, University of Maryland
The biotechnology and medical diagnostics industries could soon benefit from a technique that promises enhanced sensitivity over conventional fluorescence detection methods. Developed at the University of Maryland in Baltimore and called directional surface-plasmon-coupled emission, it shows promise for applications such as DNA hybridization, high-throughput screening for drug discovery, single-molecule detection, immunoassay and bioassay development, protein analysis and emerging nanophotonics technologies.
Surface plasmons are basically electron oscillations on the surface of metals — or, more specifically, in an interfacial region between a metal and a dielectric medium. Although usually nonradiative, they are the basis of surface plasmon resonance. This process, which is used to measure bioaffinity reactions, occurs when irradiation with photons excites the surface plasmons on the metal to radiate in a measurable way. Excitation of surface plasmons also can occur on subwavelength-thick silver, gold or aluminum films. And in some cases, placing an excited fluorophore next to the thin film will excite the plasmons to radiate into the substrate.1,2
Spatial discrimination
With directional surface-plasmon-coupled emission, researchers couple an excited fluorophore to surface plasmons on a thin film, which in turn radiates with the fluorophore’s photophysical characteristics at unique angles. The interaction occurs for fluorophores that are roughly 20 to 200 nm from the metal surface. In this region, highly p-polarized emission occurs over a narrow angular distribution, and normally isotropic emission converts into easily collected directional emission (Figure 1).
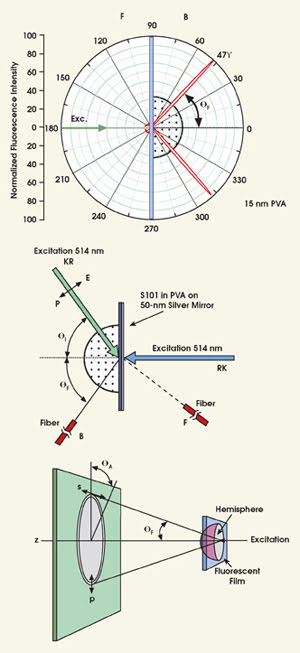
Figure 1. These results illustrate the angular distribution of S101 emission excited using the reverse Kretschmann configuration. Top: Experimental geometry for measurement of free-space emission and directional surface-plasmon-coupled emission is illustrated for Kretschmann and the reverse configuration. Middle: Emission of S101 in PVA is observed with a hemispherical prism and reverse Kretschmann excitation. Bottom: A hemispherical prism allows observation of the directional technique’s emission cone.
In both conventional surface plasmon resonance and the directional technique, researchers often use a so-called Kretschmann configuration for the optical setup. Here, the thin film rests on a high-refractive-index glass slide optically coupled to a prism by index-matching fluid. The sample is then excited through the prism.
There are variations to this setup — some more efficient than others — depending on the desired result. For example, using a reverse Kretschmann sample/optical configuration, researchers observed the angular dependence of 15-nm PVA film doped with sulforhodamine 101 (S101) on a silver substrate approximately 50 nm thick. In this configuration, though, the sample is excited from the air or sample side, which has a refractive index lower than that of the prism; direct excitation of the surface plasmons is impossible.
This is not the case with the Kretschmann setup, where incident light is completely reflected at all angles except where the incident light equals the surface plasmon angle (θsp).3 For incident angles approaching this angle, there is an evanescent wave in the air sample side, distal from the incident light. The evanescent field, which is enhanced about twentyfold by the resonance interaction,4,5 extends approximately 200 nm into the air or sample, providing a finite volume for fluorescence excitation.
On the prism side of the sample, emission is sharply distributed at ±47° from the normal axis, where the intensity observed on the sample side is much lower and not sharply distributed at any particular angle. Interestingly, the thicker S101 PVA samples showed a wider angular distribution with higher emission intensity on the front side. Such results are consistent with the lower-efficiency coupling into the surface plasmon for fluorophores more distal from the metal surface.
With typical optical components, the collection efficiency is 1 percent or less; however, by integrating the intensities observed at all angles on both the front and back sides, the University of Maryland researchers estimated that the coupling efficiencies were as much as 50 percent with directional surface-plasmon-coupled emission; the thicker samples had lower coupling efficiencies.6 The technique thus offers a range of possibilities for high-sensitivity fluorescence detection, especially when one considers that autofluorescence is effectively negligible.
Another benefit of the technique is that the emission is polarized in the plane of incidence (p-polarized) irrespective of the polarization of the excitation. This process characteristic implies that emission is due to coupling of the fluorophores and surface plasmons and suggests that the emission dipoles that are parallel to the plane of incidence couple into the surface plasmon. Those perpendicular to the plane of incidence do not cause emission — or at least they display only very weak coupling. It is possible to further reduce the impact of any autofluorescence from more distal fluorophores by collecting only the polarized component or the light emanating at the appropriate angle.
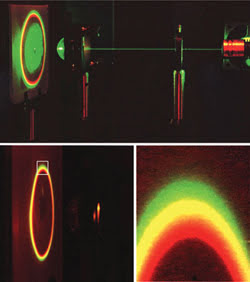
Figure 2. Directional surface-plasmon-coupled emission varies with the optical setup, as shown for a mixture of three fluorophores using reverse Kretschmann excitation and the hemispherical prism configuration. (Top, no emission filter; bottom, through a long-pass filter.)
The directional technique also is strongly wavelength-dependent (Figure 2). In essence, different emission maxima display surface-plasmon-coupled emission at different angles, with the shorter wavelengths occurring at larger angles. In one experiment, a mixture of S101, rhodamine B and pyridine 2 clearly showed the separation of the emission wavelengths with the use of reverse Kretschmann 532-nm excitation and a hemispherical prism, where the same free-space emission spectra were observable at different observation angles. This demonstrated that surface-plasmon-coupled emission can provide wavelength resolution without the process set-up requiring additional dispersive optics.
The technique also offers a choice of thin metal films, including silver, gold and aluminum, with the emission efficiency dependent on their reflectance properties (Figure 3).7 A choice of metals could facilitate the development of numerous fluorescence-sensing platforms.
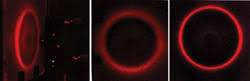
Figure 3. During directional surface-plasmon-coupled emission from an S101-doped PVA film on a 50-nm continuous gold substrate, the observation cone (side view, left/front view, middle) is somewhat wider than for silver (right), which is due to the wider range of decreased reflectivity for gold than for silver.
One area that could benefit from the technique is the measurement of DNA hybridization. This process is now central in biotechnology and medical diagnostics, where fluorescent dyes usually show an increased quantum yield, changes in energy transfer or even excimer formation upon binding to double-stranded DNA.
DNA and protein analysis
In these methods, DNA hybridization is detected by a change in the emission characteristics of the label, which occurs upon formation of the double-stranded DNA. Directional surface-plasmon-coupled emission, however, offers a new approach to DNA and protein analysis, where the intensity change is due to localization of the fluorescent label near the thin metal film by the DNA or protein binding reaction.
In this regard, the technique offers several advantages for measuring DNA hybridization or other binding reactions:
• When using the Kretschmann or reverse Kretschmann configurations, binding can be detected without a change in probe intensity caused by the binding event. This makes the technology applicable with many more probes than just those that display changes in quantum yields.
• The technique effectively rejects label fluorescence (background) more distant from the metal. This background suppression occurs by two potentially cumulative methods. First, there is decreased coupling at larger distances from the metal. Second, when using the Kretschmann configuration, fluorophore excitation occurs preferentially near the metal surface (approximately 200 nm), providing for sample spatial discrimination.
• Because the directional emission occurs over much larger distances than the size of biomolecules, researchers can place several layers of the capture molecules on the metal surface for increased sensitivity.
To demonstrate the technique’s utility for DNA analysis, the team studied the emission of Cy3-labeled DNA oligomers bound to complementary unlabeled oligomers that were themselves bound to a silver surface (Figure 4).8 In this high-sensitivity fluorescence-sensing platform, surface hybridization resulted in directional surface-plasmon-coupled emission due to Cy3-DNA into the prism whether the sample was excited with the Kretschmann or reverse Kretschmann configuration. A large fraction of the total potential emission, which is characteristic of Cy3 even though it’s the result of radiating surface plasmons, couples into the prism, thus improving sensitivity.
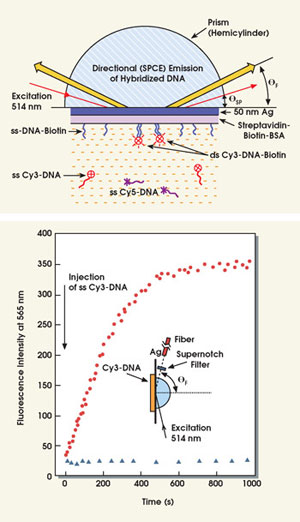
Figure 4. In the experimental setup illustrating directional fluorescence emission from hybridized DNA (top, figure not to scale), the surface-bound capture oligomer was labeled with biotin (ssDNA-biotin). The oligomer complementary to the capture oligomer was labeled with Cy3 (ssCy3-DNA). A shorter oligomer noncomplementary to the capture oligomer was labeled with Cy5 (ssCy5-DNA). When unbound, this is more distal from the silver. Researchers also observed directional surface-plasmon-coupled emission fluorescence at 565 nm (Cy3-DNA emission) upon injection of an ssCy3-DNA in the presence (•) and absence (Δ) of a complementary ssDNA-biotin deposited on the protein-coated 50-nm silver mirror.
The team’s findings indicate that, when the surface is illuminated through the prism at the surface plasmon angle, the sensitivity is the greatest because of the enhanced intensity of the resonance evanescent field. These DNA results suggest that the technique will find numerous applications for nucleic-acid and protein-binding reactions.
Another area that could benefit is high-throughput screening. Microliter plates could easily be coated with metal, especially gold, because gold’s stability is higher than that of silver and aluminum. In fact, silver or gold could be deposited on demand by light deposition prior to use in the detection process.9
Also worth exploring is the technique’s potential for single-molecule detection. Here, the total signal level is often limited by the collection efficiency and autofluorescence from the samples. The method could increase the efficiency of light collection as well as reduce background fluorescence. In addition, higher collection efficiencies could be obtained using simple shapes.
Finally, another valuable opportunity lies in combining directional surface-plasmon-coupled emission with the effects of small metal particles.10,11 Subwavelength-size silver particles can increase the quantum yields as well as the photostabilities of fluorophores within 100 Å of the surface.
In contrast, the directional technique occurs over much larger distances, but without an increase in radiative decay rate. Hence, appropriately positioned fluorophores may allow optimization of these effects, displaying a dramatically greater intensity and photostability because of the interactions of the metallic particles and the directional emission at the surface plasmon angle of the emission.
Meet the authors
Chris D. Geddes is an associate professor and director of the Institute of Fluorescence at the University of Maryland Biotechnology Institute in Baltimore. He is editor in chief of the Journal of Fluorescence, editor in chief and founding editor of Who’s Who in Fluorescence and Annual Reviews in Fluorescence, and executive director of the Society of Fluorescence.
Ignacy Gryczynski is a research professor at the University of Maryland School of Medicine.
Joanna Malicka is a research associate at the Center for Fluorescence Spectroscopy.
Zygmunt Gryczynski is an associate professor in the department of biological chemistry and molecular biology at the University of Maryland and assistant director of the center.
Joseph R. Lakowicz is director of the center. He is the founding editor of the Journal of Biomedical Optics and the Journal of Fluorescence, co-founder and co-president of the Society of Fluorescence, and co-editor of Who’s Who in Fluorescence and Annual Reviews in Fluorescence.
Acknowledgments
This work was supported by the National Center for Research Resources.
References
1. J.R. Lakowicz (2004). Radiative Decay Engineering 3: Surface Plasmon-Coupled Directional Emission. ANALYT. BIOCHEM., 324(2), pp. 153-169.
2. I. Gryczynski et al (2004). Radiative Decay Engineering 4. Experimental studies of surface plasmon-coupled directional emission. ANALYT. BIOCHEM., 324(2), pp. 170-182.
3. J.R. Lakowicz et al (2003). BIOCHEM. BIOPHYS. RES. COMM., 307, pp. 435-439.
4. T. Liebermann and W. Knoll (2000). Surface plasmon field-enhanced fluorescence spectroscopy. COLLOID SURFACES, 171, pp. 115-130.
5. K. Kurihara and K. Suzuki (2002). ANALYT. CHEM., 74, pp. 696-701.
6. A. Van Orden et al (1998). ANALYT. CHEM., 70(7), pp. 1444-1451.
7. I. Gryczynski et al (2004). Surface plasmon-coupled mission using gold films. J. PHYS. CHEM. B. Submitted.
8. J. Malicka et al (2003). DNA hybridization using surface plasmon-coupled emission. ANALYT. CHEM., 75(23), pp. 6629-6633.
9. C.D. Geddes et al (2003). LANGMUIR, 19, pp. 6236-6241.
10. C.D. Geddes and J.R. Lakowicz (2002). J. FLUORESCENCE, 12(2), pp. 121-129.
11. J.R. Lakowicz (2001). ANALYT. BIOCHEM.,
Key Benefits of Directional Surface-Plasmon-Coupled Emission
According to Chris D. Geddes and colleagues at the Center for Fluorescence Spectroscopy at the University of Maryland in Baltimore, directional surface-plasmon-coupled emission offers new opportunities for high-sensitivity fluorescence detection based on the following features:
• Spatial discrimination. Only fluorophores in proximity couple into the metal surface and provide directional emission.
• Excitation discrimination. Using Kretschmann excitation, only fluorophores within 200 nm of the surface are excited by the evanescent field.
• Polarization discrimination. The coupled emission is p-polarized irrespective of both the mode and the polarization of the excitation.
• Spectral resolution. Different fluorescence emission maxima display directional surface-plasmon-coupled emission at different angles.
• A choice of metal films. This gives researchers the flexibility to employ various chemistries and/or metal stabilities and their optical properties.
The technique is technically simple, requiring only the preparation of thin metal films.