Determining laser damage thresholds and the automatic characterization of laser damage in tissue may enable safer and more effective treatment of patients with skin conditions in the future.
Florian Treiber, Gloria Seidel, Alexander Kabardiadi-Virkovski, Peter Hartmann, University of Applied Sciences Zwickau
Lasers are currently used in many skin care applications, both medical and cosmetic. However, it is important to ensure proper handling and monitoring of the laser source to avoid damage to the skin tissue; in these applications, the laser beam is usually applied directly to the skin. Typical laser treatments that would occur in a clinic, dermatologist’s office, or cosmetic studio include skin tightening, skin resurfacing, tattoo removal, or targeted removal of malignant skin lesions. The power of the laser ranges from a few watts to 100 W.
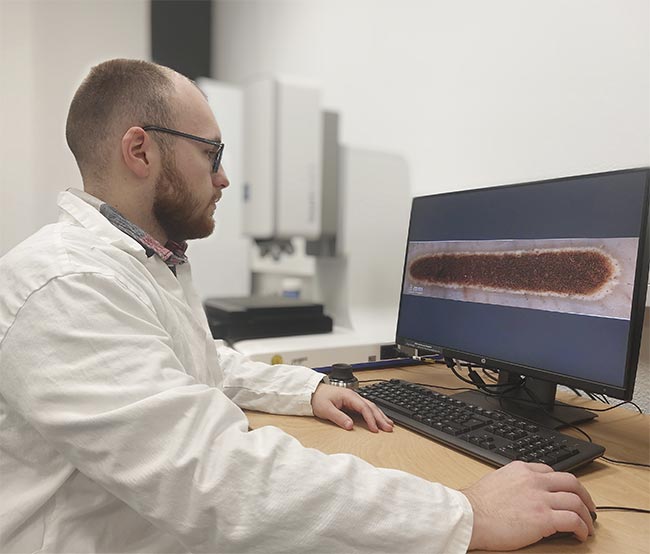
Florian Treiber oversees the microscopic imaging and processing of skin damage from a laser. Courtesy of Ivonne Mallasch/Fraunhofer IWS.
Improper application of laser radiation to skin tissue can cause redness, burns, scarring, or even charring of the tissue. The effects of excessive energy on skin tissue are shown in Figure 1. However, a literature review does not provide context on such skin damage or at what point such damage occurs, due to the lack of research that has been performed. The following study outlines a basic procedure to obtain explicit damage thresholds that can serve as a foundation for future laser skin treatment.
Building a baseline
To prevent skin damage, it is crucial to understand the type and extent of damage that occurs at different energy threshold values (Figure 1). However, limited information exists to quantify these values, as revealed in a search of relevant publications covering laser-tissue interactions. Therefore, it is essential for researchers to collect data to establish a foundation for future experiments on acceptable power in laser treatments as well as an approach to a consistent evaluation of skin damage that is incurred. Furthermore, a public database of images of such burns can be launched.
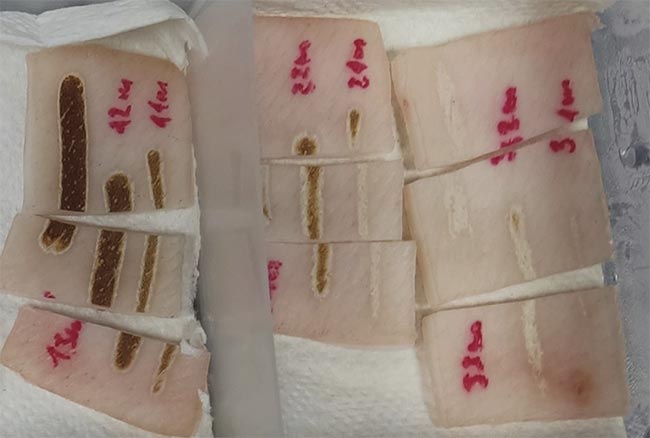
Figure 1. A section of possible resulting damage from a CO2 laser. Courtesy of Florian Treiber/WHZ.
It is widely recognized by clinicians that improper use of laser radiation can cause significant harm to patients. However, documentation on specific values, such as laser damage thresholds, for treatments among a representative group of potential subjects is limited. The skin’s structural inhomogeneity often leads to significant fluctuations in results between patients.
To gain a better understanding of how lasers could affect the skin of patients, it is crucial to establish clear boundary conditions for testing to enable reproducible results. In the authors’ study, pig skin was used as a substitute for human skin. A carbon dioxide (CO2) laser with a working wavelength of 10.6 µm was selected as the test laser due to its frequent use in dermatology to remove scars and wrinkles, in tumor surgery for a more precise removal of cancer cells with simultaneous coagulation of the blood vessels, and in dentistry for the removal of the frenulum of the tongue or for the treatment of sensitive tooth necks1.
Difficulty in obtaining data
Generalizing the knowledge gained from observing the interaction of different laser systems with different samples is difficult due to a number of variables, including the scattering in the tissue, which depends on the cellular structure. Therefore, the results and findings are valid only under previously defined framework conditions.
The extent of tissue damage caused by laser skin applications is determined by both biological factors and device parameters. Biological factors include skin type and tissue structure at the irradiated site2. The thickness of human skin varies in different parts of the body, so it is expected that areas with thicker skin will experience different levels of damage compared with those with thinner skin. When considering device parameters, the wavelength used is of utmost importance3. The chosen wavelength is important to maintain because different wavelengths interact with tissue in varying ways and penetrate to different depths4. Additional important device parameters include the irradiation duration, irradiation power, and operating mode of the laser system during the course of a procedure3.
Pig skin for simulation
During the study, pig skin was selected as a simulation material for human skin due to its high similarities to human skin in the composition of both the epidermis and the dermis5. Additionally, the skin regrowth and healing in pigs is comparable to that of humans. Although there are differences in the structural level of the subcutis and the pH value of the skin, the experiments were conducted within 24 h on the skin of a Piétrain sow6. No animals were killed for this work.
Laser-induced skin damage usually occurs during movement rather than under static conditions. While the applicator is moved by the treatment staff, if the patient feels any pain, their instinct is to move. To simulate a moving process, a test setup that generated damage in the form of a line was created.
Figure 2 displays the experimental setup, with the CO2 laser beam source visible on the right-hand side. The laser beam was directed onto the sample surface, and a lens above the sample was used to vary the beam diameter on the surface. The sample was placed on a holder mounted on a linear axis to simulate movement at varying speeds.
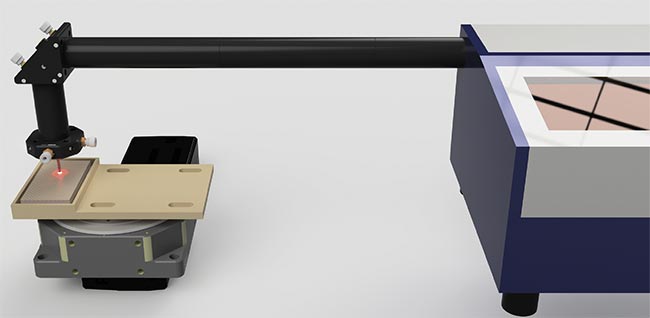
Figure 2. An experimental setup to assess laser damage. Courtesy of Florian Treiber/WHZ.
The setup was used to vary the beam diameter on the sample surface. Additionally, the power of the laser and the speed of the linear axis were adjusted accordingly. Table 1 provides a complete list of the experimentally determined test parameters, based on a power range typically used in a medical context.
Table 1. Experimentally Determined Parameters

By varying the test parameters, 45 lines of damage were created on the sample surface with the laser. Figure 1 displays the appearance and consequences of this skin damage.
To evaluate and analyze the damage using the developed semiautomated evaluation routine, it must first be digitized. For this study, digitization was performed using a digital microscope. After capturing the image, a scale was added to the damage image, which is necessary for further calculations (opening image).
To accurately describe the extent of damage in relation to power and time, it is crucial to differentiate between the affected areas. In this study, tissue discoloration as a structural feature for rapid damage assessment was selected as a means for differentiation.
A total of three thermally damaged zones were identified (Figure 3). The most severe damage area was defined as damage area 3. This section pertains to the region enclosed by the green contour in Figure 3, where the laser radiation directly contacted the skin surface. Therefore, the interaction between the laser radiation and the skin surface is most prominent in this area. The discoloration of the thermally damaged region is characterized by severe burning of the skin cells, resulting in a dark brown to partially blackish discoloration.
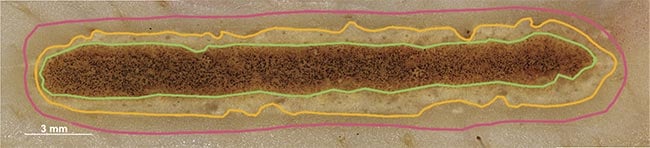
Figure 3. Characterization of one of the damaged areas by the thermal discoloration that has occurred. Courtesy of Florian Treiber/WHZ.
Damage area 2 exhibits a beige- to sand-colored discoloration between the orange and green contours (Figure 3). It is believed that the collagen fibers in the skin have contracted to create this effect. The extent of damage in this area is dependent on the heat transfer in the tissue from damage area 3 and the influence of the laser beam’s edges. However, the laser beam’s damage potential is reduced since it has the highest energy in the center. Toward the outer edges, the energy decreases and is therefore not sufficient to cause severe burns.
The final visible area of damage is located between the pink and orange contours, which was declared as damage area 1. The structural feature is a slight shadow on the tissue, and the damage is exclusively due to heat transport in the skin.
This study investigated various methods for classifying these three areas, and the software classification program AnyLabeling was found to be the most effective7. After selecting the desired image area (damage area), this program automatically segmented the entire area using artificial intelligence in conjunction with other image processing mechanisms, such as edge detection. However, it is important to note that this method had its limitations and some areas of damage still needed to be segmented manually — when energy output was low and when only slight damage occurred, producing edges that were not clear.
Semiautomated calculation
After segmenting all the laser damage to the skin samples, the next step was to determine its extent. To accomplish this, a semiautomatic evaluation routine was developed (Figure 4). This routine was used to calculate the surface energy and area of the damage.
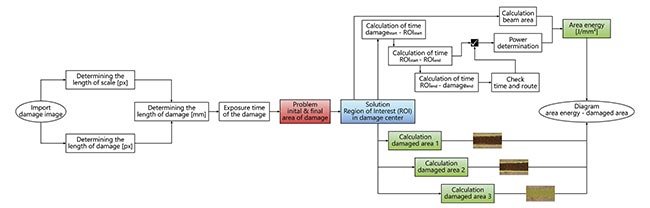
Figure 4. An evaluation routine to calculate the damaged area and the surface energy input. Courtesy of Florian Treiber/WHZ.
In this study, the laser was manually switched on and off. To facilitate damage comparison, a region of interest (ROI) was defined at the center of each contact area with a constant length. This length was used to calculate the individual damage areas and the energy per unit area.
Before entering the measured values into the diagram, the authors created the theoretical curve (Figure 5a). The lower plateau in yellow represents the area where only slight damage occurs at low energy values. As the surface energy increases, the damage gradually increases. Once the damage threshold value is reached, the damage area can then be recategorized to the next level of intensity. Here, a plateau is formed where no further significant increase in the damaged area can be observed. The discoloration was chosen as a baseline characteristic for categorization. Hence, the damage after the structural change of the tissue due to burning and the one-time setting of the discoloration only varies in its extent up to the maximum size given by the blasting area. Anything beyond the beam diameter or length of the line damage was caused by heat transport in the tissue.
In practice, a progression corresponding to the previously explained theory was demonstrated (Figure 5b). To achieve this, damage areas 2 and 3, i.e., medium and severe burns, were presented consecutively in a diagram. It is evident that damage does not occur up to a surface energy of 1.5 J/mm². Beyond this value, the extent of the burn increases rapidly and eventually reaches the upper plateau.
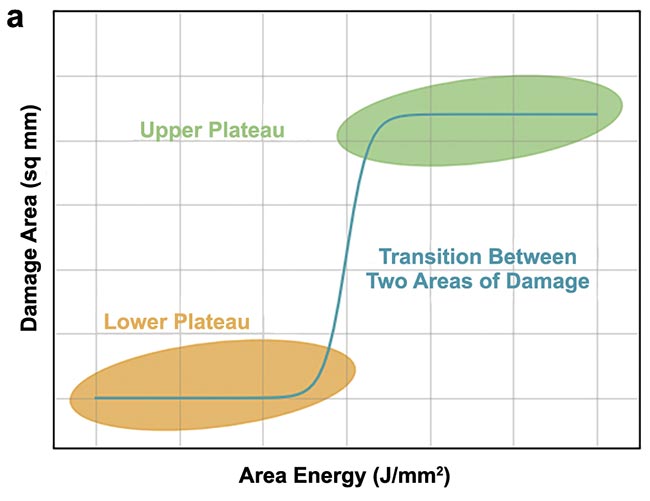
Figure 5. An expected (a) and practical (b) course of the damaged area over the area energy (green dots with an offset of 1.8 J/mm2).
Courtesy of Florian Treiber/WHZ.
Future testing
The purpose of this study was to establish a foundation for future experiments aimed at determining threshold values. These findings will enable the planning and execution of explicit and detailed experiments for different wavelengths, facilitating the generation, collection, and evaluation of scientific data related to laser safety. Future studies will target 1064-nm laser irradiation — which penetrates deeper into skin than CO2 lasers — as well as compare preservation methods and resulting damage.
Consequently, existing laser safety regulations could be validated or revised, as needed. In the future, an assessment by the authors’ team will determine the volume of damage from the affected area. Other techniques for recording and assessing damage are also being tested. The artificial intelligence used for segmentation is being further trained and improved.
Meet the authors
Florian Treiber received his bachelor’s degree in biomedical engineering in 2023. He is currently studying medical and health technology at the University of Applied Sciences in Zwickau, Germany. In his research, he is currently working on laser burns of the skin and their evaluation; email: [email protected].
Gloria Seidel completed her studies with a diploma degree in physical engineering in 2008 at the University of Applied Sciences in Zwickau, Germany, with a specialization in biomedical engineering. She is laboratory engineer in the laboratories for optics, laser technology, and physics at the same university; email: [email protected].
Alexander Kabardiadi-Virkovski graduated with a bachelor’s degree from Westsächsische Hochschule Zwickau in 2013 and a master’s degree in nanotechnology in 2015. His research focuses on optical metrology, with notable work on wavefront analysis and AI methods in imaging. Since 2015, he has been a research associate at Fraunhofer IWS, AZOM department, and became deputy group leader in 2020. He is currently pursuing a Ph.D. in collaboration with Friedrich Schiller University Jena and University of Applied Sciences in Zwickau, Germany; email: [email protected].
Peter Hartmann received his Ph.D. from Friedrich Schiller University Jena. In 1992, he joined the Institute of Optics and Quantum Electronics Jena. He is currently a professor of optical technology at the University of Applied Sciences in Zwickau, Germany, and head of the Fraunhofer Application Center of Optical Metrology and Surface Technologies; email: [email protected].
References
1. H.J. Eichler and J. Eichler. (2015). Laser, 8th ed., Springer Vieweg Berlin, Heidelberg.
2. C. Raulin and S. Karsai. (2013). Lasertherapie der Haut, 1st ed., Springer Berlin, Heidelberg.
3. C. Schneeweiss et al. (2020). Leitfaden für Fachkundige im Laserschutz, 1st ed., Springer Spektrum Berlin, Heidelberg.
4. M.A. Abduljabbar et al (November 2022). Treatment of facial telangiectasia using long-pulsed Nd: YAG laser. AIP Conf Proc, Vol. 2660, No. 1.
5. J.O. Archambeau and G.W. Bennett. (1984). Quantification of morphologic, cytologic, and kinetic parameters of unirradiated swine skin: a histologic model. Radiat Res, Vol. 98, No. 2, pp. 254-273.
6. W. Meyer. (1996). Comments on the suitability of swine skin as a biological model for human skin [in German].
Hautarzt, Vol. 47, No. 3, pp. 178-182.
7. V.A. Nguyen. (n.d.). AnyLabeling — Effortless data labeling with AI support. Retrieved February 2, 2024, https://github.com/vietanhdev/anylabeling.