Silicone materials resist yellowing better than UV-curable acrylates or epoxies.
Randall Elgin, Lightspan Application Laboratory, and Bill Riegler and Rob Thomaier, NuSil Technology LLC
Dramatic improvements in the performance of LEDs have placed fresh demands on the encapsulation materials used to package these devices. Advances in LED operating efficiencies and output performance have resulted in single devices that can produce as much as 100 lm/W. These diodes operate at junction temperatures in excess of 125 °C and require encapsulation materials that surround and protect them without degradation and that protect the entire package from excessive thermomechanical stress.
In these devices, the encapsulation materials are part of the light path that couples the light output of the semiconductor substrate to the outside environment. Therefore, optical clarity is a requirement, and it is critical that the encapsulation materials maintain consistency during an LED’s expected 10,000-h operating lifetime. This requirement has prompted the evaluation of encapsulation materials that can perform at a higher optical level than ever.
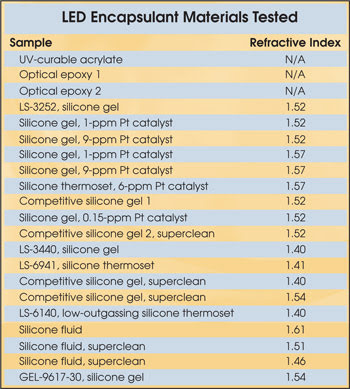
The material selected to meet this task is typically silicone, which is a versatile material with high temperature performance and a variety of elastomeric properties, including flexibility. Silicone can provide excellent clarity as well as refractive indices from 1.40 to 1.61. Its initial use in low-power LEDs has been extremely successful, but as the demands of the industry have grown (particularly with the advent of white LEDs and the expansion of the operating wavelengths of the semiconductor substrates into the UV range), all types of encapsulation materials are required to meet a new level of performance.
LED packages juxtapose many types of materials and operate at high temperatures and light flux densities that cannot be easily simulated. To evaluate some of the most common encapsulation materials and their response to UV, a comparative study was performed using several optically clear epoxies, acrylates and silicones (see table). This study tested encapsulation materials isolated from any potential material incompatibilities present in a typical operating device and measured the effect of UV on the encapsulant only.
Materials were evaluated for their change in optical transmission resulting from a 680- to 6800-J/cm2 dose of UV radiation. Included in the silicone samples were gels and thermosets: A silicone gel is a soft material with a durometer measurement of hardness of Type “OO” (similar in consistency to Jell-O brand gelatin), while a silicone thermoset is a harder, rubbery material with a durometer measurement of Type “A” (similar in consistency to a pencil eraser).
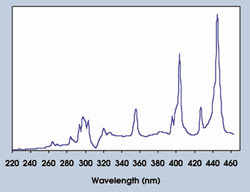
Figure 1. To test the effects of UV radiation on LED encapsulant materials, exposure to ~190 mW/cm2 was provided by a 400-W visible (indium) bulb, producing the above spectrum.
Samples were prepared by curing the test material between microscope slides spaced 2 mm apart with a silicone gasket. The UV radiation exposure was provided by a 400-W visible (indium) bulb, also known as a “V” spectrum bulb (Figure 1). Samples were placed 6 in. from the bulb, where the total UV intensity was nominally 190 mW/cm2. Temperatures during the exposure ranged from 75 to 90 °C.
Degradation caused by the UV radiation was measured by comparing the transmission spectrum before and after exposure (Figure 2). Exposure to UV always causes the transmission spectrum to shift down and to the right. In other words, it causes the roll-off, or the knee of the curve, to move further into the visible part of the spectrum. The amount of this shift is equal to the amount of sample “yellowing” as seen by the naked eye.
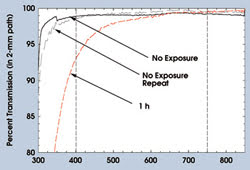
Figure 2. UV-related degradation of an encapsulant was measured by comparing the transmission spectrum before (left) and after (right) exposure. UV exposure causes the spectrum to shift down and to the right.
UV-related degradations in the light transmission of acrylates and epoxies — where transmission is <80 percent at 400 nm — are visibly yellow. Degradations in transmission in a typical silicone are barely visible to the naked eye and require a spectrophotometer to determine relative transmission loss (Figure 3).
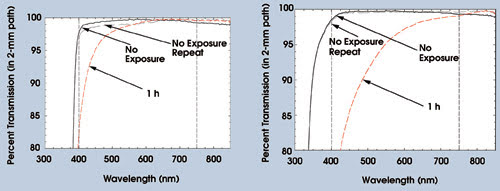
Figure 3. Changes in transmission of a UV-curable acrylate (left) and an epoxy (right) appear after 1 h of exposure to UV radiation.
Possible causes of yellowing
There are several curing mechanisms for silicones: amine, peroxide and addition cures. Of these, the most appropriate for optical applications is the addition cure. Addition-cure systems require a catalyst in amounts measured in parts per million. This requirement sometimes makes these systems vulnerable to cure failure because any contaminant that neutralizes the catalyst effectively prevents the silicone from curing. Using larger amounts of catalyst to ensure curing, however, is known to cause considerable yellowing when exposed to elevated temperature.
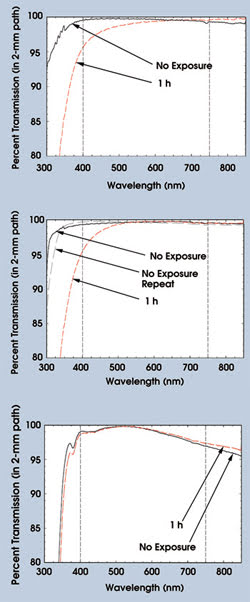
Figure 4. A comparison of various silicone materials with refractive indices of 1.40 (Top) 1.52 (middle) and 1.57 (bottom) demonstrated that the 1.57 material clearly had the least amount of degradation under UV radiation.
In the study, a variety of catalyst concentrations were evaluated — from 0.15 to 9 ppm. Over this range, there was no discernible difference in the transmission degradation from UV exposure. Two different catalysts also were tested, with no discernible difference between them.
The refractive index of a silicone is controlled by the amount of phenyl in the side-chain position on the silicone polymer. Developments in the use of silicones for optical encapsulation had created a perception that higher-refractive-index silicones are more prone to yellowing. This was tested, and the results do not support this idea (Figure 4).
Also evaluated were samples considered extremely low in impurities. The samples either were manufactured to be superclean by taking precautions during the synthesis or were sent out for postsynthesis supercritical extraction. Among those tested from this group were materials that covered a variety of refractive indices.
For many reasons, cleanliness and low contamination levels are desirable, but the data do not show this to be a yellowing source. Superclean materials do not consistently demonstrate enhanced resistance to yellowing compared with materials manufactured through normal laboratory processes.
Clues to the cause of yellowing
For some materials in this study, the rates of yellowing change caused by UV exposure for up to 10 h were evaluated. During this test, samples were monitored every 3.3 h for their change in transmission (Figure 5). Interestingly, some materials, such as LS-3252, showed the greatest degradation in the first 3.3 h, but subsequent exposures did not cause further appreciable degradation. This suggests that the cause of the yellowing is exhausted early.
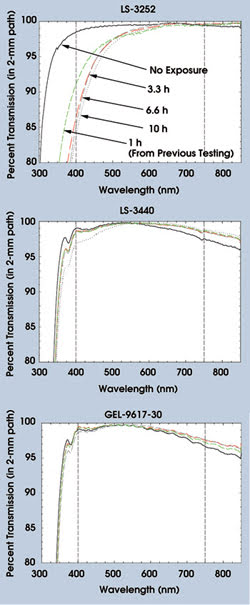
Figure 5. To test the rate of yellowing caused by UV exposure, samples were monitored every 3.3 h for changes in transmission. Some materials, such as LS-3252 (top), showed the greatest degradation in the first 3.3 h, but subsequent exposures did not cause additional falloff.
Also, certain materials showed almost no change in transmission from the beginning. These include LS-3440 and GEL-9617-30, which received 10- and 6.6-h exposures, respectively.
In an effort to understand the yellowing mechanism, the study focused on the silicone encapsulants and tried to use a “one cause, one effect” approach. However, the cause of yellowing in silicone encapsulants proved to be not so simple, and the yellowing mechanism is more likely a complex chemistry involving multiple-system interaction.
UV light is energetic enough to break molecular bonds and create ions, electrons and activated molecules. A device designer’s first defense against yellowing is to select a material that is transparent to the wavelength of interest. This selection process becomes increasingly difficult starting at about 400 nm. With decreasing wavelength, the options are increasingly limited, as all materials eventually will reach a breakdown point.
Our investigation rests with the results of the 10-h exposures, and the materials that performed innately well, in an effort to determine the factors that provide UV-degradation resistance. This has led to insights regarding the nature of the silicone curing mechanism itself as well as to an appreciation for the intense energy these encapsulation materials experience. This constitutes a new design criterion to meet in the development of materials for high-brightness LED packaging.
Encapsulation and packaging once was considered an easy part of device design. Semiconductor designers have been so successful that they have created a need for novel materials that can withstand intense radiation levels. Silicones may provide a solution to this demanding problem.
At this point, improvements in withstanding UV radiation appear to lie in the material’s chemical design. The challenge is to develop chemistry that can coexist with the energy output of today’s LED packages.
Meet the authors
Randall Elgin is a senior applications engineer at NuSil Technology LLC’s Lightspan Application Laboratory in Wareham, Mass.; e-mail: [email protected].
Bill Riegler is product director of engineering materials and Rob Thomaier is R&D director, both at NuSil’s headquarters in Carpinteria, Calif.; e-mail: [email protected].