By coupling two technologies, researchers find new applications in nonlinear optical microscopy and endoscopy.
Min Gu, Swinburne University of Technology
During the past 16 years, nonlinear optical microscopy has evolved from a photonic novelty to a well-established laboratory tool. Rapid development of fiber optic components with growing functionalities and decreasing size provides enormous opportunities for innovations in nonlinear optical microscopy.
A team of researchers at Swinburne University in Victoria, Australia, and at the University of Florida in Gainesville have coupled nonlinear fiber optic microscopy with microelectromechanical systems (MEMS) mirrors to achieve three-dimensional endoscopic imaging of breast cancer tissue and of intestinal epithelial cells and intestinal crypts in vitro.
At present, in vivo imaging and long-term bedside studies by nonlinear optical microscopy have been limited because of the lack of a compact nonlinear optical instrument/imaging technique. Considerable work has gone into the miniaturization of nonlinear optical microscopy. However, major challenges remain to creating a nonlinear optical endoscope that can function within internal body cavities. These include the efficient delivery of the excitation beam and the nonlinear optical signals, the flexibility and compactness of the probe, and the miniaturization of its laser-scanning mechanisms.
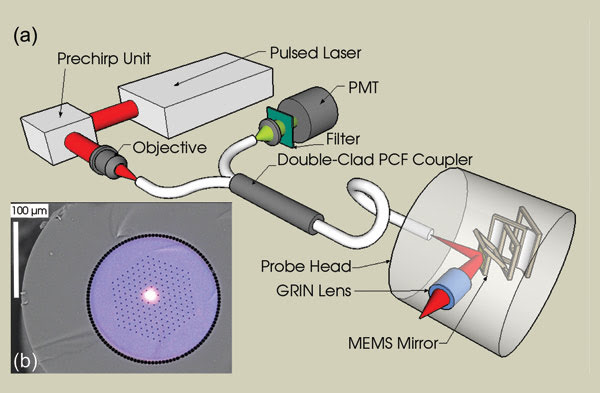
Figure 1. (a) The double-clad photonic crystal fiber (PCF) is shown as part of a nonlinear optical endoscope system (b). A cross section of the double-clad fiber uses femtosecond pulsed 800-nm near-infrared single-mode illumination confined in the central core and visible 410-nm multimode propagation in the inner cladding overlaid (PMT = photomultiplier tube; GRIN = gradient index).
The researchers have overcome these challenges to produce both optical fiber-based nonlinear microscope and endoscope systems based on single-mode optical fiber or double-clad photonic crystal fiber, a MEMS mirror and a gradient index lens (Figure 1). As the double-clad photonic crystal fiber in a nonlinear optical microscope is used to deliver near-infrared laser beam excitation and to collect nonlinear optical signals in the visible range, it is important to understand the properties of the fiber under various operating conditions.
A double-clad photonic crystal fiber is used to enhance the sensitivity of the endoscope system as its large core alleviates nonlinear optical effects during pulse delivery in the near-infrared wavelength range and high numerical aperture for signal collection in the visible wavelength range. The detection efficiency of the nonlinear optical microscopy system based on double-clad photonic crystal fiber is approximately two orders of magnitude higher than that based on a standard single-mode fiber.
The unique properties of microstructured photonic crystal fiber also unlock alternate imaging modalities. The polarization-preserving nature of double-clad photonic crystal fiber enables endoscopic second-harmonic-generation polarization anisotropy measurements, a useful tool in the determination of biological structure order. The performance of these measurements is limited by the depolarization of the second-harmonic-generation signal by the microstructures of the inner cladding.
The all-fiber separation of the excitation and nonlinear optical signals has been achieved by a double-clad photonic crystal fiber coupler. The coupler efficiently discriminates the laser excitation and nonlinear optical signals with little loss, ~1 dB, and a splitting ratio of 97:3. This is sufficient for 3-D two-photon fluorescence and second-harmonic-generation imaging in conjunction with a gradient index rod lens.
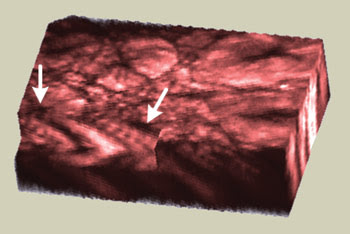
Figure 2. A 3-D reconstruction of a rat large intestine is shown. The arrows indicate intestinal crypts.
Huikai Xie, associate professor at the University of Florida, has simplified endoscopic design and packaging by incorporating a MEMS mirror in the scanning unit. MEMS optical scanners for endoscopic biomedical imaging applications scan large optical angles at a high speed with minimal electrical requirements. The MEMS mirror is based on electrothermal actuation and can perform wide-angle optical scans over 60° from a less than 12-VDC supply. The large scan range, low-voltage operation and small size render the MEMS mirror suitable and safe for clinical applications.
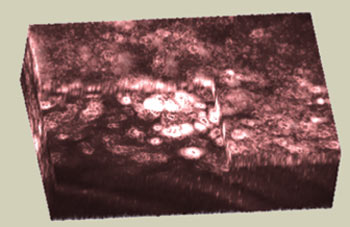
Figure 3. A 3-D reconstruction shows human u-87 MG glioblastoma breast tissue from in vitro image slices.
The amalgamation of these results has enabled the first demonstration of 3-D high-resolution tissue imaging through a fiber optic miniature nonlinear microscope (Figures 2 and 3).
Meet the author
Min Gu is professor of optoelectronics and director of the Centre for Micro-Photonics at Swinburne University of Technology in Victoria, Australia; e-mail: [email protected].