Optical fibers are now being used throughout the global communications network. And as the ensuing demand for greater data capacity grows, they are being installed at ever increasing rates.
MARIE FREEBODY, CONTRIBUTING EDITOR
Fiber optics technology plays a critical role within the telecommunications sector, thanks to the wide-scale and growing adoption of the technology in communication and data transmission services. Optical fibers are used in high-speed data transfer services, in both short- and long-range communications. Combined with the added surge in cloud-based applications, audio-video services, and video-on-demand technology, the market shows no signs of slowing.

Holey fibers could offer a means to increase bandwidth with lower latency, improved power handling, and improved quality of light transmission by replacing conventional glass cores with air or a vacuum to produce hollow-core “light pipes.” Courtesy of the Optoelectronics Research Center, University of Southampton.
Today, the insatiable appetite for data means that demand for optical fibers is booming. Installation has never before been so prolific. This is true within both established markets, such as the U.S., and in markets such as China, where there is massive growth.
In addition to long-distance deployment, over the last few years fiber has continued to penetrate deeper into the network, with fiber end points edging ever closer to homes, businesses, and cell towers. Indeed, in some countries, fiber is now being deployed directly to the home, boosting premium broadband access speeds in countries including Japan and Korea to the Gb/s level.
As the power of the internet grows — and with it our internet usage — there are huge pressures on existing bandwidth.
“The bandwidth demands on our
internet-enabling communication networks have surged to a point where the high bandwidth and data communication speed capabilities of optical fiber are needed everywhere,” said Merrion Edwards, director of development of optical fiber and cable market and technology at Corning Inc. in Corning, N.Y. “Legacy copper and microwave communication systems are constantly being upgraded to fiber.”
A particular feature of recent change has been the deployment of fiber for backhaul, and more recently fronthaul, in mobile communications networks. In a landscape where microwave systems were once the traditional workhorse, the new trend in fiber is expected to accelerate as mobile technology moves toward high-speed 5G services. In the coming years, 5G networks will herald further large-scale deployment of fiber. In these networks, fiber will be used to collect signals from arrays of remote antennas and deliver them to compute nodes for signal processing and recovery — referred to as the mobile fronthaul. The resulting processed signals are then sent onward to other users through the core network, which is referred to as the mobile backhaul.

Researchers fabricate a microstructured fiber preform in the Optoelectronics Research Center at the University of Southampton. Courtesy of the Optoelectronics Research Center, University of Southampton.
Fiber is also being deployed at an ever-increasing rate both within and between data centers. The majority of the world’s data traffic actually flows within data centers rather than on the supporting external core networks.
Data transmission with SDM
The primary focus over the past 10 or so years has been on developing higher spectral efficiency optical data signals for transmission on existing fiber infrastructure, known as digital coherent transmission. Globally, the research community is looking at improved ways of transmitting signals through fiber by better exploiting the spatial domain. This can be done either by better uses of arrays in existing single-mode fiber, by integrating multiple spatial channels through the same fiber by incorporating more cores in the fiber cross section, or by exploiting multiple modes in a multimode fiber. These techniques are referred to as space division multiplexing (SDM).
“The ultimate goal of this research is to deliver a greater amount of data at greater speed and, most importantly, at a significantly reduced cost per transmitted bit than currently possible,” said David Richardson, professor, deputy director, and head of advanced fiber technologies and applications in the Optoelectronics Research Center (ORC) at the University of Southampton in England.
One of the main challenges with SDM relates to demonstrating the killer application and presenting a compelling business case sufficient to persuade operators to move away from simply installing ever more parallel systems based on single-mode fiber technology. Beyond that, Richardson notes that a huge effort is needed to make the technology practical and manufacturable at an acceptable cost.
“In reality,” he said, “each of these steps represents a major hurdle, and we may just need to satisfy ourselves with laying more of the same, albeit with better integrated and more energy-efficient components and subsystems.”
Tackling the challenge from many angles includes introducing smaller 200-µm outer diameter optical fibers, which have a 30 percent reduction in cross-sectional area, relative to traditional legacy fibers with a 242-µm outer diameter, while retaining the same 125-µm glass core diameter. This opens the door to a whole new range of smaller and denser microcable designs that are addressing the problem of duct congestion in access networks.
Optical transmission data rates have been steadily increasing since the birth of the industry in the 1980s. Where early transmission speeds were measured in Mb/s, today’s single wavelength transceivers run at 200 Gb/s, which, within a dense wavelength division multiplexing (WDM) system, enables the transmission of multiple Tb/s per fiber.
“The most significant change in optical fiber for communications in recent years has been the advent of ITU G.654.E ultralow attenuation, large effective fibers designed for use in terrestrial longhaul networks,” Edwards said. “Leveraging learnings from the advanced high-capacity solutions used in transoceanic submarine systems — the high-power handling, low attenuation, and low nonlinear coefficient characteristics of the new large-effective-area fiber — for terrestrial applications can enable operators to move to [more than] 200-Gb/s single wavelength systems while retaining the regenerator-to-regenerator reach of their existing network. These fibers are enabling tomorrow’s speed with today’s footprint.”
Hollow-core fibers
Another potential approach to improving data speed and capacity is the development of hollow-core optical fibers. Today’s conventional optical fibers, used the world over, contain a glass core at the center
of the fiber through which light is transmitted. However, not only does this glass center limit the speed of the light as it passes through, it also adversely affects other aspects of its propagation, ultimately limiting the performance of the fiber
and the associated optical system.
The ORC will lead a £6.1 million (€6.9 million; $7.8 million) EPSRC (Engineering and Physical Sciences Research Council)-funded program to develop the next generation of fiber optics, with a view to position the U.K. as a world leader in this emerging technology.
The new program will investigate and explore the performance limits of hollow-core fiber technology. It will also develop new ways of manufacturing, as well as methods of interconnecting, these new optical fibers to more conventional fiber types and other optical components, such as lasers. Dubbed Airguide Photonics, its goal is to replace the conventional glass core with air or a vacuum, to produce
hollow-core “light pipes.” By guiding light through air, rather than through glass, these new optical fibers offer increased bandwidth with lower latency, improved power handling, and improved overall quality of light transmission.
Although this is an exciting avenue of research, some experts — including Joris Van Campenhout, program director of Optical I/O at research center imec in Belgium — believe that standard single-mode fiber will remain the medium of choice for most high-end fiber optic communication applications, given its cost and performance metrics.
This is echoed by Edwards, who admits that hollow-core fibers are an interesting concept, but one that is still very much in the early research phase. “While they offer interesting prospects in terms of lower latency transmission, their very high attenuation and cost, and the challenge of making them in meaningful lengths, makes them currently impractical for application in today’s communication systems,” she said.
Optical interconnects
Another hot topic includes the advent of cost-reduced silicon photonic technologies, which has enabled the rapid migration to 100-G optics, with 400 G expected in just a few short years.

A researcher checks a microstructured fiber preform in the Optoelectronics Research Center at the University of Southampton. Courtesy of the Optoelectronics Research Center, University of Southampton.
Van Campenhout has witnessed increased levels of photonic integration, particularly within optical interconnects, which help to reduce power but increase the bandwidth of these optical components.
“Integrated photonics, both on indium phosphide and silicon substrates, are gaining traction over incumbent technologies based on discrete optics,” he said. “Intradatacenter optical interconnects are increasingly driving the volume deployment of fiber optic transceivers.”
While silicon photonics offers an enticing solution to reduce the losses suffered in coupling optical components, Van Campenhout notes that for silicon photonics, low-cost laser integration remains a technical challenge. Further reductions in packaging costs are also an issue.
It is widely believed that we are reaching the fundamental physical limit of single-wavelength optical transmission system data rate and reach — known as Shannon’s law. With so many avenues of research to explore, advances will likely come from many sides. According to Edwards, the big long-term challenge for the industry will be how to serve the needs of the capacity hot spots, typically the east-west inter-region data center interconnect links that have very high annual traffic growth rates, while delivering low cost-per-bit solutions.
“It is thought that advances outside of increasing the data rate further are required,” she said, “such as an expanded transmission spectrum, using both C+L band, and SDM.“
Protecting Security in Optical Fibers
One of the world’s major players in quantum cryptography has recently demonstrated secure communication over 500 km of optical fiber. The Cambridge Research Laboratory of Toshiba Research Europe in Cambridge, England, has devised a new protocol for quantum key distribution (QKD) — the work was published in Nature in May 2018. The report details a new approach to extend the range to over 500 km of standard telecom fiber.
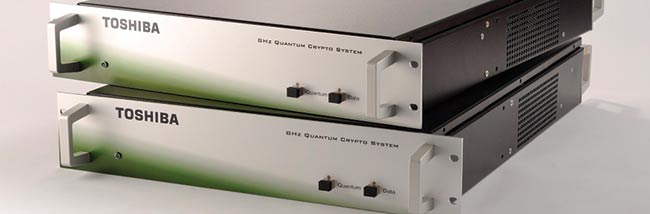
Toshiba’s prototype quantum key distribution (QKD) system allows key distribution over standard telecom fiber links exceeding
100 km in length and bit rates sufficient to generate 1 Mb/s of key material over a distance of 50 km — sufficiently long for metropolitan coverage. Courtesy of Toshiba Research Europe.
Until now, the typical range of QKD has been limited to a few hundred kilometers of optical fiber. This is because the photons carrying the information can be scattered and thereby lost from the fiber, reducing the rate at which secret keys can be formed. The new advance, called twin-field QKD, enables sensitive data — such as bank statements, health records, and digital identity — to be transmitted in optical networks between cities. Unlocking such personal information requires a “key,” which is encoded upon a single photon transmitted through an ordinary optical fiber. Any attempt to read the photon alters its encoding, which allows the secrecy of each key to be tested and guaranteed.

Optical fiber manufactured from a Corning facility. Courtesy of Corning Inc.
Unlike other existing security solutions, quantum cryptography is secure from all future advances in mathematics and computing, even from the number-crunching abilities of a quantum computer. It is therefore expected to be an essential tool for protecting communication infrastructure from cyberattacks in the future.
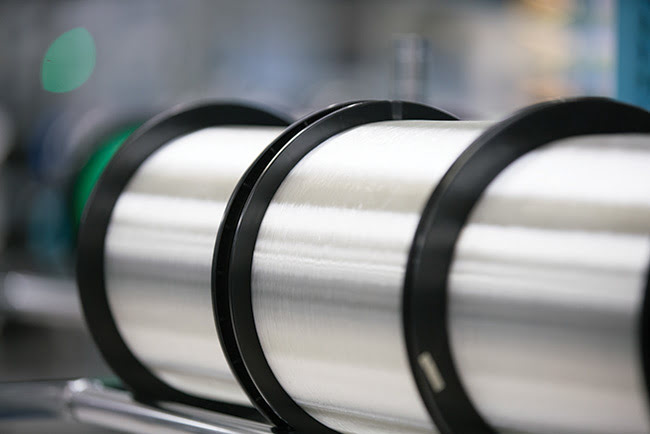
Corning created the first low-loss optical fiber for telecommunications. Courtesy of Corning Inc.
In conventional QKD, single photons are typically sent from one end of the fiber to the other. In contrast, for twin-field QKD, light pulses are sent from both ends of the fiber to a central location, where a photon is detected. Provided it is impossible to tell which end of the fiber the photon came from, this technique effectively doubles the transmission distance at a given rate.
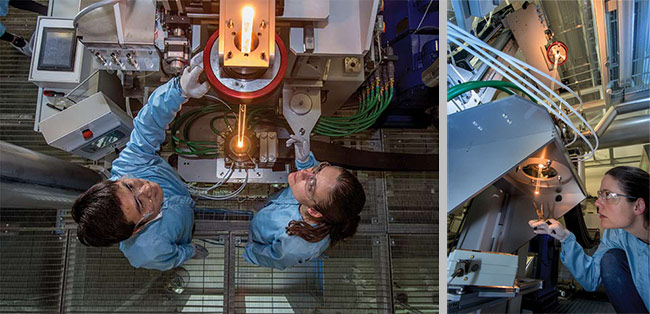
Researchers in the Optoelectronics Research Center (ORC) at the University of
Southampton demonstrate a live fiber draw. Courtesy of the Optoelectronics
Research Center, University of Southampton.