Frank Bernhardt, Fraunhofer Institute for Production Technology IPT
Bypassing the time-consuming wear stages of heating and cooling allows quick testing of mold coating lifetimes while increasing efficiency for precision glass molding.
Optical components that fulfill complex functions by using complex-shaped surfaces – for example, free-form surfaces for laser beam shaping optics – are a target of current developments in optics design and production (Figure 1). Such high-precision glass lenses can be manufactured economically only by replicative techniques. The process of precision glass molding is the gold standard for the fabrication of these high-quality lenses. In precision glass molding, glass preforms are placed between molds and heated up in a vacuum. After the system is heated to the molding temperature of 400 to 650 °C, the glass is pressed firmly for several minutes until the mold form is homogeneously filled with glass. After controlled cooling, the pressed lens has the desired optical and geometric properties, with no need for postprocessing steps.
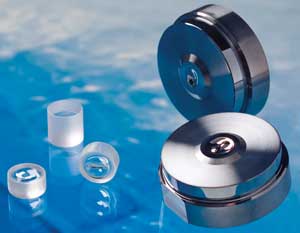
Figure 1. Molding tools with free-form surface molded lenses for laser applications.
The process efficiency and, therefore, the cost depend strongly on the service lifetime of the molding tools. Protective coatings on the molds significantly reduce thermochemical reactions, corrosion processes or other functional degradation mechanisms that result in, for example, glass sticking (Figure 2). But the coatings must fulfill a multitude of requirements that can be categorized in mechanical, thermal and chemical aspects, or combinations of these. Mechanical loads are exerted – e.g., by pressure on the glass gob or by friction between the mold’s surface and the glass – that can result in mold deformation, glass breakage or scratches. Therefore, hard materials such as cemented tungsten carbide are used as the mold material and diamondlike carbon or ceramics as their coating materials.
Diamondlike carbon coatings additionally are known to show low friction in various tribological systems, which can reduce mechanical loads. Obviously, thermal loads are present in the glass molding process, so all materials involved must withstand several hundred to 1000 thermal cycles. In addition, the coefficients of thermal expansion of the mold, the coating and the glass should be similar to reduce stresses and friction in the contact zones or in the coating itself.
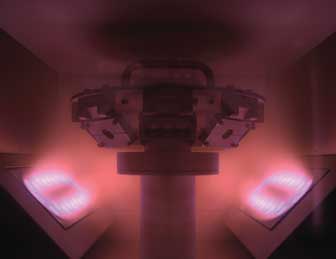
Figure 2. Coating process: sputtering cathodes and sample holder.
The chemical load is due mainly to undesired reactions such as adhesion of glass on the coating, diffusion processes in the coating and corrosive attack at the coating surface, all of which are strongly temperature-dependent. These loads affect mainly the surface quality of the coated molds. The thermochemical reactions – i.e., the chemical reactions induced specifically by the temperature influence – are the most critical reason for early end-of-service lifetime by the loss of the coating-protective functionality.
Although many investigations have been undertaken to understand and prevent undesired change of the coatings’ surface integrity, every new glass type poses a new challenge to the coating design: After all, each glass has a different composition and is molded under unique conditions and therefore interacts differently with the coatings.
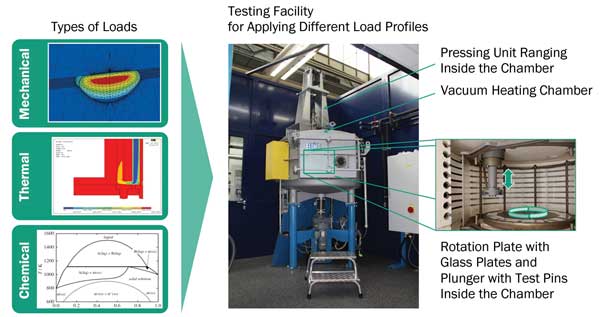
Figure 3. Working principle for high-throughput performance testing of glass mold coatings.
However, the ultimate goal in coating development is the functional validation of the improved protective properties. These properties and their functional degradation time are decisive for the service-lifetime assessment. Relevant negative alterations of high-performance coatings that lead to failure of the protective properties appear only after several hundred to 1000 pressing steps, so such investigations have been very time-consuming and costly.
As a result of extensive manual quality assurance needed during testing, multiple pressing tests block valuable machine time and keep personnel from actual production. The total costs for lifetime analysis often mean that production begins without a guarantee of success.
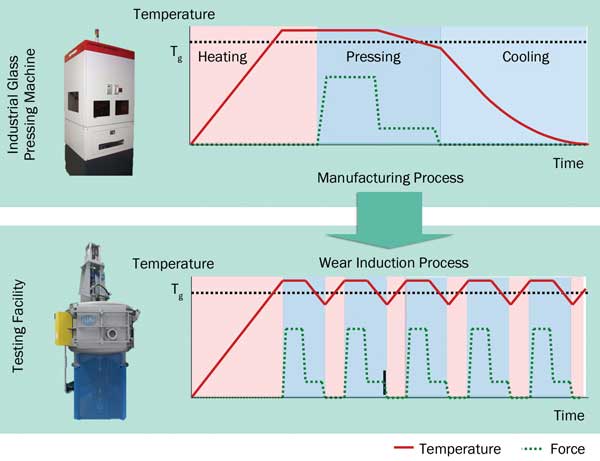
Figure 4. Concept of defined load application and realization by testing facility design.
Until recently, there was no quick test for performance evaluation on an improved or newly developed coating. But now, Fraunhofer Institute for Production Technology IPT has developed quick tests for the precision glass molding process. IPT’s testing facility assesses the service lifetime of mold coatings for precision glass molding in an efficient way. The facility is specifically designed to induce the same loads and load combination as in the real process, but to bypass the time-consuming stages of heating and cooling – immaterial to the wear – thereby significantly accelerating the testing procedure (Figure 3).
By applying process simplifications and a high degree of automation, the facility provides a versatile investigation platform. Since no ultrahigh positioning accuracies are needed, in contrast to the actual lens manufacturing process, the machine’s design can be simplified and adjusted to test tools rapidly. In contrast to other evaluation techniques, all relevant loads on the coating are applied simultaneously during testing, just as in the real process; if the loads are applied separately using different testing methods, no information about their interactions can be gained. This machine can execute 120 fully automated process-equivalent pressing steps in a day; in comparison, a production glass molding machine can produce about 25 lenses at a time – a process requiring constant manual intervention (Figure 4).
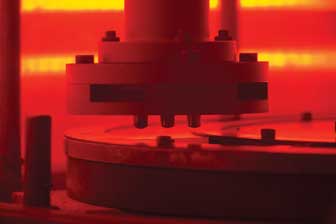
Figure 5. Three spherical pins just before contacting the glass samples during a testing facility run.
The testing facility consists mainly of a heating vacuum chamber, a pressing unit and a sensor system. The vacuum chamber can be heated up to 800 °C and evacuated down to less than 2 mbar. Alternatively, the process can take place in an inert nitrogen atmosphere. Instead of glass gobs, glass plates are used in this machine. These are placed on a rotating table inside the chamber. The coated mold equivalents (“pins”) are mounted to the pressing unit ranging inside the chamber, perpendicular to the glass plates. The pin holder can be equipped with up to three pins that can be tested simultaneously regarding their material performance (Figure 5).
After several hundred or 1000 pressing steps, which need multiple full-load runs of the test cycles without changing the pins, the pins can be analyzed with regard to the thermochemical wear. To do this, the coated test pins are pressed into the glass under controlled atmosphere, force, temperature and position conditions resembling the process loads best possible under minimal time and manual work effort. Compositional changes and changes in the coatings’ or hard materials’ microstructure as well as fracture at the coating or glass preforms are the main aspects of interest here.
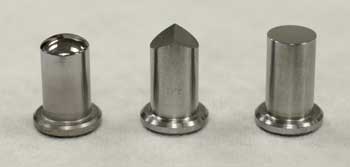
Figure 6. Set of test pins for simulating different molding conditions in fabrication of free-form and diffractive lenses.
In addition, different pin surface geometries are currently available, opening the opportunity to analyze the contour dependency of the mold wear. This becomes more and more interesting for the increasing complexity in advanced optics, with regard to the replicative manufacturing of free-form, high-curvature or diffractive lenses (Figure 6).
To demonstrate the applicability of the test facility, different validation tests have been conducted. Pressing tests have shown a good fit with simulation predictions of glass behavior in the facility (Figure 7). This is not self-evident, since the homogeneous heating of the large chamber volume by heating rods is very different from IR lamp heating of a specimen in a much smaller cavity, standard in industrial glass molding machines. Primarily, the high heating dynamics of IR lamps are not needed in the long period between heating up to process temperatures of 450 to 650 °C and cooling down to room temperature again.
Figure 7. Profile of test pressing in the facility (left) and simulation results (right).
The sample throughput rate can be optimized by increasing the number of pressings per full run, instead of by reducing the heat-up time. The indirect, radiation-based heat transfer from IR lamps is strongly dependent on the absorption properties of the mold and glass materials at the irradiated lamp frequencies. IR heating has some disadvantages, since the homogeneity of heating cannot be assured when different materials involved in the pressing assembly must be heated up isothermally – to the same temperature for both the mold and the glass.
At the testing facility, the heating of the samples and the glass is implemented by convection through the process atmosphere and through broadband radiation. Therefore, the adjustment of equal temperatures at the molding die and throughout the glass preform can be ensured. Another benefit is that chalcogenide glasses (e.g., for night-vision systems in automotive or other mass-developed civil applications) also can be heated and molded in this facility. Because they are transparent in the IR spectrum, these glass types were difficult to heat up in standard glass molding machines.
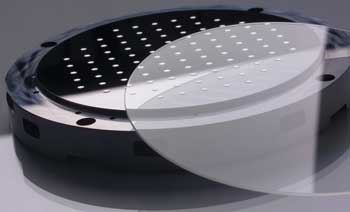
Figure 8. Coated wafer mold for microlens fabrication.
Preliminary results also have shown that the wear of the test pins after the pressing cycles resembles the characteristics of the wear known from production environments. Future research and development efforts at Fraunhofer IPT will target the standardization of the testing procedure and the validation of the testing process for different application ranges. Furthermore, the knowledge-based investigation of influential parameters regarding the degradation of the mold coating function will improve understanding of coating design (Figure 8).
Meet the author
Frank Bernhardt is a research associate at Fraunhofer Institute for Production Technology IPT; email: [email protected].