Telecentric and standard area backlights are powerful tools in locating and measuring edges, while in-line and ringlight illumination sources excel in applications in which evaluating surface detail is critical.
JEREMY GOVIER, EDMUND OPTICS
For machine vision applications requiring telecentric lenses, the proper illumination can be critical to the success of the imaging system. Achieving properly angled, even illumination can greatly affect the contrast of the image and the accuracy of measurements calculated from the image.
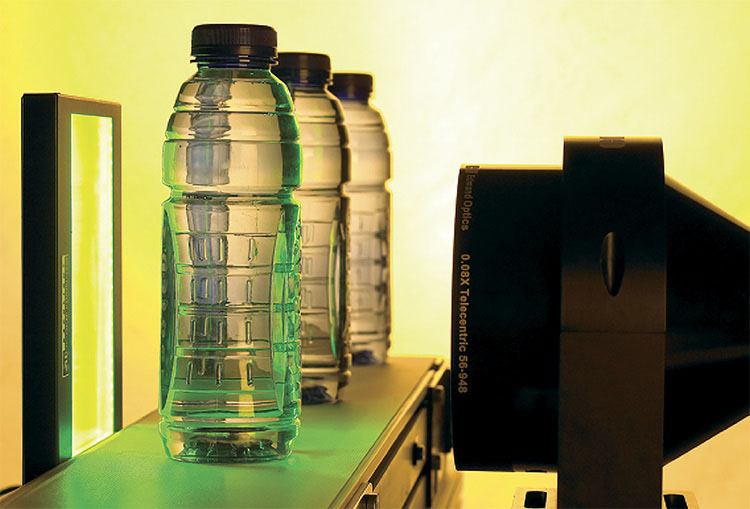
A telecentric lens and backlight illuminator used for bottle inspection. Courtesy of Edmund Optics.
To understand how illumination interacts with a telecentric lens, it’s important to first understand what makes a telecentric lens different from a standard imaging lens. Telecentric lenses are primarily used in gauging applications in which an object’s size or the location of its surface features is measured. The telecentricity of the lens allows for magnification to be accurately calibrated, regardless of whether the working distance between the lens and object is changed. The unique properties of telecentric lenses can also be advantageous for imaging objects with certain structures — such as well plates, tubes, or pins — that are perpendicular to the object plane. In these applications, the object’s position in the field of view does not change how these structures will appear to the lens (Figure 1).
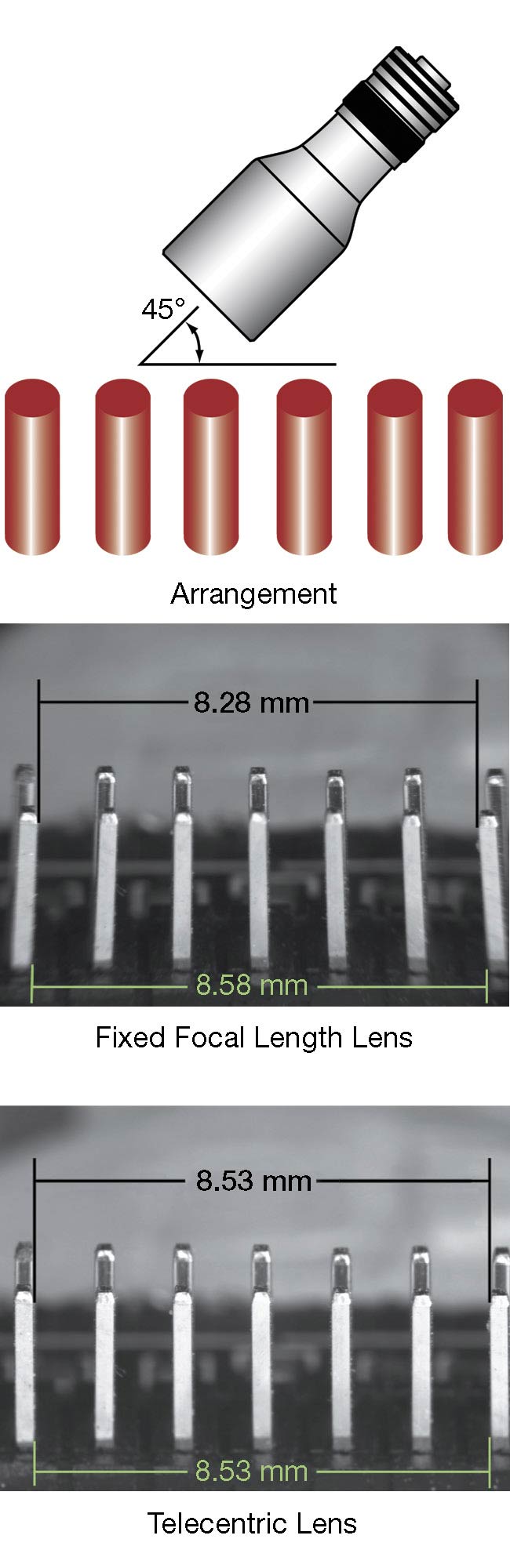
Figure 1. Objects such as jumpers on a circuit board that are imaged by a standard lens will appear different depending on their position in the lens’s field of view, while they will appear identical to a telecentric lens no matter where their location is inside the field of view. Courtesy of Edmund Optics.
Bear in mind that a telecentric lens has an angular field of view of zero, meaning that no matter the working
distance, the field of view of the lens remains the same. In a standard lens, as the object is moved closer or farther from the lens, the field of view will change as a function of the angular field of view (Figure 2).
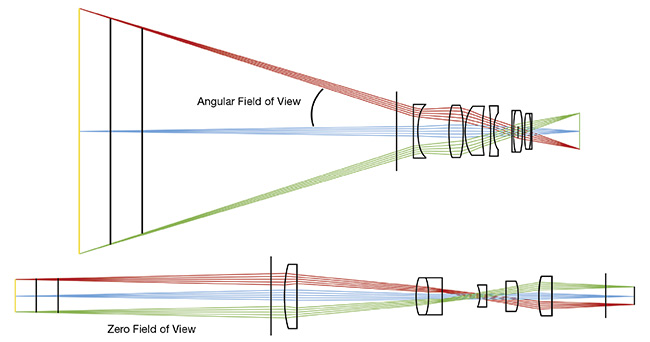
Figure 2. The angular field of standard lenses results in parallax error (top), while the zero angular field of view of telecentric lenses eliminates this issue (bottom). Courtesy of Edmund Optics.
In more technical terms, this change occurs because the chief ray angles
for all the field points are parallel (Figure 3). This means that the rays at the
center of each cone of light that the lens receives from the object all enter
at the same angle; all of the cones of light point in the same direction.
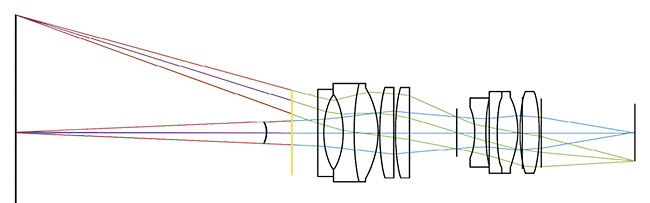
Figure 3. The angle between the marginal rays defines the numerical aperture of the lens. Marginal rays (red) and chief rays (purple). Courtesy of Edmund Optics.
Chief and marginal rays
In addition to chief rays and center rays, marginal rays also help to explain the effects of illumination. The rays on the outside of the bundle that indicate
the bundle’s width are known as marginal (Figure 3). They reveal how much light is collected from the object, and they affect parameters such as the depth of field of the lens. Descriptions of the numerical aperture and the f/# of a lens refer to information about the marginal rays.
Generally, though not always, telecentric lenses have low numerical apertures in object space, meaning that the marginal ray angles are relatively shallow. This is because many telecentric applications require large depths of view that can only be achieved with small numerical apertures. Another reason for telecentric lenses to typically have shallow marginal ray angles is the need to keep the size of the front optics from growing too large. Since the chief rays are all parallel, the front optics must be at least as large as the field of view because the chief rays do not change height after leaving the lens. The degree to which the lens must be larger than the field of view is a function of the marginal ray angles and the working distance. The higher the marginal ray angle, the higher the ray will need to strike on the lens, increasing its size. Since longer working distances are often a requirement of a telecentric
lens, the numerical aperture is the only parameter that can be adjusted to reduce the lens size (Figure 4).
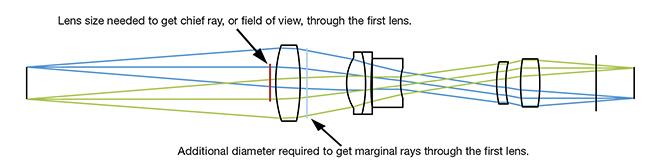
Figure 4. Demonstration of the effects of numerical aperture on the size of the first lens element. Courtesy of Edmund Optics.
Backlight illumination
The most common type of lighting
for telecentric lenses is backlighting,
because when measuring the shape of an object or the location of through holes, the best way to get a high-contrast image is to silhouette it. With backlighting, edges are easy to find and the high contrast allows for accurate measurement of their position. The drawback of this approach is that surface detail information gets lost because the surface itself is shadowed. While backlight illumination is great for locating and measuring edges, it is less than ideal for measuring or distinguishing surface features such as printed text. Two common ways to back-illuminate an object are with a standard area backlight and a telecentric backlight.
A standard area backlight uses a large diffuser to spread light from one or multiple sources over the entire area of the field of view. The light travels through a very wide diffusion angle via the diffuser. One advantage of area backlights is that they are compact and take up little space. They are also inexpensive compared with alternative illumination sources. The wide diffusion angle makes for simple alignment of the light, with no need to accurately tilt the light source with respect to the lens. A large portion of the illumination does not make it into the numeric aperture of the telecentric lens, but it is also relatively easy to increase the amount of illumination going into the diffuser to make up for this waste.
The wide diffusion angle of the backlight has one particular disadvantage that can be detrimental in gauging applications: It can cause light to strike features on the tops of parts, causing scatter from these surfaces that makes it into the lens. This is particularly noticeable if the part edge is a cylinder or if it has a bevel. This scatter will cause the edge of the silhouette to have lower contrast and will therefore shift the location at which an edge-find will detect the edge away from its true location (Figure 5a).
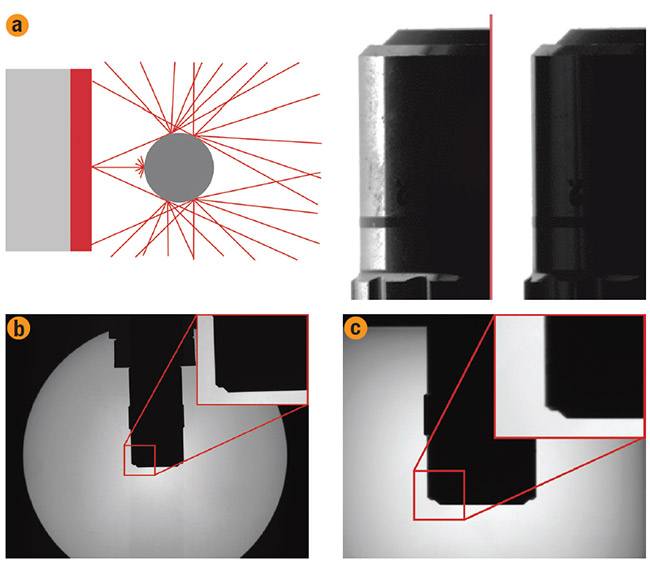
Figure 5. Depiction of light
rays scattering off the edges of an object and of blurry edge contrast on the silhouette of an objective
using a conventional backlight (a).
Depiction of sharp edge contrast on the silhouette of an objective using a telecentric backlight (b). Depiction of slightly blurrier edge contrast on the silhouette of an objective using a collimated area backlight (c). Courtesy of Edmund Optics.
To solve this edge scatter effect, the wider diffusion angle rays must be prevented from leaving the illumination
and striking the surface of object. To
prevent this, a form of collimated backlighting called telecentric illumination is required, from which results a finite beam of light with a small diffusion angle. The angular extent of this diffusion is a little more than the marginal ray angles of the telecentric lens used to observe the object. This way, the telecentric lens’s numerical aperture is completely filled, but only a few extra rays that could scatter off the object are sent out, greatly reducing the edge scatter effect that is seen with a standard area backlight (Figure 5b).
However, the difficulties in using a telecentric backlight must be weighed against the gains in eliminating the edge scatter. Telecentric backlighting systems are very large because the collimating lenses are about the same size as the telecentric lenses, both in diameter and length. To evenly illuminate the field of view, the lenses must be larger than the field of view. The length of the illuminator, when added to the length of the telecentric lens — plus the working distance — can end up extending over 1 m in many cases, making it difficult to fit into an assembly line.
With the size increase comes a cost increase because large lenses and
barrels drive the costs of illuminators to be considerably higher than for a standard area backlight.
The small diffusion angle, which is just a little larger than the numerical aperture of the telecentric lens, means that care must be taken in aligning the illuminator to the lens to capture the light. Even small misalignments result in no light getting into the system. On the one hand, it is an advantage that the small angular extent of the illuminator means fewer LEDs are needed to drive the illuminator; this slightly reduces costs. On the other hand, the limit on the number of LEDs also constrains the intensity of the illuminator.
If the size or cost of the telecentric
illuminator is too large for a given application but edge scatter is still a concern, options are available that offer a compromise between the telecentric
illuminator and the previously mentioned backlights. Special backlights called collimated area backlights are smaller and cheaper and allow for wider diffusion angles than a telecentric illuminator, but still less wide than a standard area backlight. Collimated area backlights use thin structured illumination components to reduce the
divergence to around 10° to 20° — much less than a standard LED backlight but more than a telecentric illuminator, which is typically on the order of single-digit degrees. Collimated area backlights can be an ideal trade-off between size and functionality among the options listed above (Figure 5c).
In-line and ringlight illumination
For applications that require illuminating the surface detail instead of silhouetting the edges, two common options for telecentric lenses exist:
in-line illumination and ringlight illumination.
With in-line, the illumination is folded into the lens using a beamsplitter. The objective lenses of the telecentric are used to collimate the light onto the
object, collect the light off the object, and image the object onto the sensor.
The use of in-line illumination is ideal for inspecting specular objects. This form of illumination is compact — it mostly uses space already taken up by the imaging lens. It places nothing additional into the working distance between the lens and object, and it adds nothing behind or around the object. In-line illumination is particularly convenient for lenses with a short working distance, in which it is difficult to get illumination between the lens and object.
One reason in-line illumination is particularly useful for telecentric lenses is that it provides bright-field illumination. The light shined onto reflective objects reflects directly back into the lens, making the objects bright, while diffuse objects — which scatter light over a wider angle — appear dimmer. This bright-field illumination can be used to get better contrast off specular and diffuse objects (Figure 6).
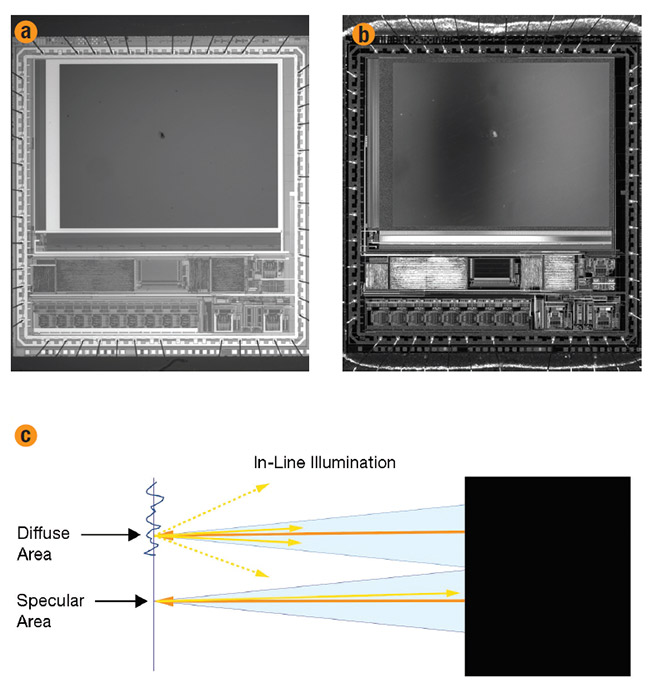
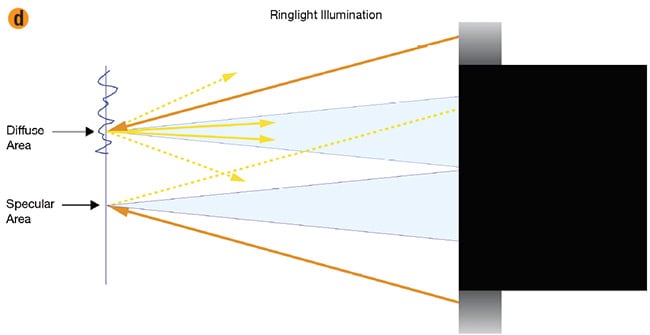
Figure 6. Depictions of images captured using a telecentric lens and in-line illumination (a) and ringlight illumination (b). In-line illumination (c) versus ringlight illumination (d). The blue cones show rays that can be imaged by the telecentric lens, the orange lines show the rays that are emitted from the illuminator,
the solid yellow lines show rays that make it back into the lens, and the dashed yellow lines show the rays that do not make it back. Courtesy of Edmund Optics.
Ringlight illumination is similar to in-line and can be used as a compromise by adding it to a lens that is not designed for in-line illumination. LEDs placed around the front of a lens and pointed at an object can generate an even illumination with little shadowing.
In-line illumination is nearly perpendicular to the object plane, which reduces shadowing of surface features. One major limitation of this type of illumination is that it must be designed into the lens. But not all designs have the space to fit a beamsplitter. The beamsplitter itself can add aberration to the system and affect telecentricity if the design has not properly compensated for it. Therefore, the use of in-line illumination is not an option for some lenses.
Another drawback is that stray light is inherent in their design and will always be present. This light bounces off the surfaces of the beamsplitter and optics and reflects back to the sensor, without ever hitting the object. Stray light can cause a general haze in the image and reduce the contrast. It can be minimized in the design, but it can never
be completely eliminated.
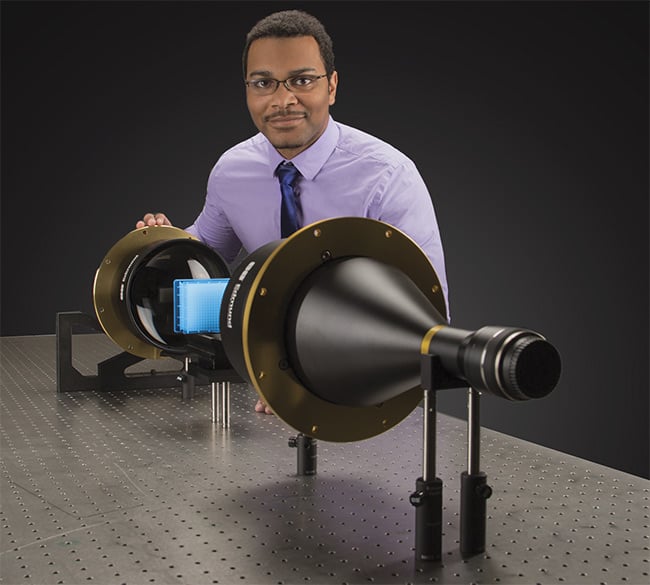
Jaylond Cotten-Martin, vision solutions engineer at Edmund Optics, pairs a telecentric lens with a telecentric backlight illuminator for high-edge-contrast imaging. Courtesy of Edmund Optics.
Ringlight illumination is similar to in-line and can be used as a compromise by adding it to a lens that is not designed for in-line illumination. LEDs placed around the front of a lens and pointed at an object can generate an even illumination with little shadowing. Unlike in-line, however, ringlight illumination is not truly bright-field, and if the light is reflected off a specular object it will not be reflected directly into the lens. For this reason, ringlights work better for making diffuse objects bright while specular objects remain dimmer.
For large telecentric lenses, ringlights can also get very large and add to the overall size of the system. And for lenses with short working distances, the angle at which the LEDs evenly illuminate the object can become steep and cause problematic shadowing.
The right choice in illumination will depend on the object being illuminated, the information that is required, and space and cost constraints. Achieving even illumination that provides enough intensity and contrast for a given application can be the key to a successful telecentric machine vision system.
Meet the author
Jeremy Govier is the principal engineer at Edmund Optics’ Tucson office. He has over two decades of experience, and he previously held positions as applications engineer, Custom Product Group manager, and director of design. He has a graduate certificate in project management from the New Jersey Institute of Technology and a bachelor’s degree in optics from the University of Rochester.