Containment, software, training and other precautions allow embedded or enclosed lasers to move into new applications.
Hiding your light under a bushel isn’t always a bad thing, contrary to the advice in the old proverb. A case in point can be found in lasers, where low-cost systems are increasingly more powerful – and, therefore, potentially more dangerous.
Using techniques and technology that effectively hide light away, however, these powerful lasers can be embedded in systems safe for use in the open by the general public. Although safe, embedded lasers face challenges as they run up against the drive to make tools that are smaller, lighter and more flexible.
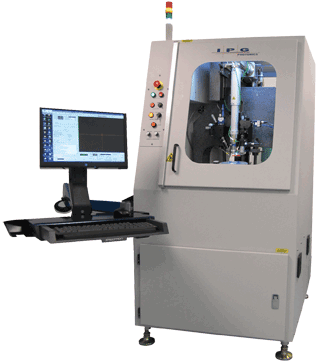
Light from a UV laser feeds up through a sealed beam delivery tube (light colored tube in center) through a focusing objective and to a workpiece. A window allows viewing of the process. The IPG Photonics IX-255 system provides Class 1 safety. Photo courtesy of IPG Photonics.
Classification, containment
Lasers are categorized according to their ability to do damage, with Class 1 products considered safe at all times under all normal-use conditions.
At the other extreme, Class 4 products can burn the skin, blind eyes or ignite materials. But, with engineering, even dangerous lasers can be rendered benign.
“Classification is based on human access to laser radiation during operation of the product,” said Jay Parkinson, a certified laser safety officer and president of Phoenix Laser Safety LLC. “CD/DVD players have embedded Class 3B or 4 lasers but are Class 1 laser products.”
This feat is pulled off by ensuring that the more hazardous levels of light from the beam cannot leave the product housing. In general, the class of an embedded laser will drop through the use of protective coverings as well as safety interlocks that shut the beam off if the housing is opened. There also may be carefully placed viewing windows and specially designed optics. A final tool in the safety arsenal is the use of scan-failure safeguards. These ensure that the beam doesn’t stop moving due to a breakdown in the mechanism that moves it from place to place. For example, scan-failure safeguards can keep a beam from dwelling too long on spots not designed to handle a stationary laser.

A laser marker with an embedded Class 4 laser inside is a Class 1 system, thanks to engineered safeguards. Photo courtesy of Laser Institute of America.
IPG Photonics Inc. of Oxford, Mass., makes fiber lasers from milliwatt output up to systems topping out in the tens-of-kilowatts range. The company has been designing and supplying laser-based systems for more than 20 years.
Its Microsystems Div. makes workstations for machining holes and other small features. These systems are Class 1 or Class 4, which can be considered closed- or open-beam. While the first category can operate safely in open spaces, the second requires safety precautions such as beam containment in temporary enclosures, warnings, interlocks and operation by trained personnel equipped with personal safety gear.
Such requirements can be a burden
to those running the laser, but may be unavoidable. Manufacturers, after all, have throughput, quality and cost targets to hit, and these targets dictate laser characteristics.
“In general, the laser process is set by the machining requirements, and the workstation is designed to enclose the light and protect personnel. It is very rare that a process would deviate from an optimal manufacturing process rather than engineer the proper safety enclosures, interlocks and beam handling devices,” said John Bickley, the Microsystem Div.’s director of sales and marketing.
The power in a beam can be substantial, and the future promises even more powerful lasers – and this trend can be seen in the offerings from laser companies. IPG Photonics, for instance, has supplied commercial lasers up to 100-kW output.
The effect of growing the power of enclosed lasers may be particularly evident in metal cutting and welding. These and other power-intensive applications may have multikilowatt lasers mounted on robot arms, which present a particular challenge.
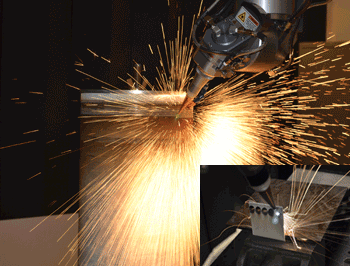
With engineering, lasers powerful enough to drill through metal during fabrication of aerospace parts can be used safely. Photo courtesy of IPG Photonics.
“As those things go up in power and complexity, and you have longer and longer focal lengths, you have virtual light sabers on the ends of robots. It can get pretty complex to contain them,” said Thomas J. Lieb, certified laser safety officer and president of Elk Grove, Calif.-based L-A-I International.
Software, wavelength, pulse width
Although advances in technology have made some containment more challenging, other innovations have made the job easier; e.g., in times past, laser safety experts had to worry about robots malfunctioning, with one possibility being that the beam would end up stationary, or almost so, on a containing wall. One solution to such a case would be multiple walls, under the theory that the extra protection would afford time to notice the error and shut down the machine.

A robot holds a beam end-effector, with the laser (not shown) making welds. Rendering safe high-power lasers in systems with long focal lengths is challenging, particularly when such systems are mounted on robot arms. Photo courtesy of L-A-I International.
In the past few years, however, licensing safety bodies have approved safety software, which enables protections that prevent this from happening. The software also keeps maintenance or other personnel from overriding the system and thereby forcing it to act in unintended ways.
Other trends that affect safety have to do with wavelength. A decade or so ago, according to Lieb, industrial applications were dominated by CO2 lasers operating in the far-IR, well outside the retinal hazard region. Now, more powerful beams are showing up in the near-IR, say from 700 to 1400 nm. Such light is invisible but presents a burn hazard; also, eye damage is possible from low-level reflections. Consequently, people exposed to such lasers may end up with a dead spot in an eye without ever feeling a thing. That puts a premium on making sure that those working with or even strolling by such systems cannot encounter the beam.
That’s one reason why major automakers and their suppliers insist that all laser systems as delivered be Class 1 devices. That requirement is a consequence of applying current standards, when the applicable documents are read in total, Lieb noted.
Industrial lasers also are operating at shorter pulse widths. Doing so can yield more precise cuts with much more limited damage to surrounding material. That and the lower cost of the technology are some of the reasons why there has been a move of industrial lasers into the picosecond- or femtosecond-pulse-width regimes.
Exposure levels
These technology changes are taking place while the acceptable exposure level is being reconsidered. Dr. David H. Sliney is now a consultant, but was formerly the program manager for laser/optical radiation at a US Army Center at the Aberdeen Proving Ground in Maryland. He is currently shepherding a revision of the applicable standard, IEC 60825-1, through the approval process. It should be published soon, he said.
The update has changes that affect embedded lasers and safety considerations. For instance, the new revision changes how repetitive pulses are tabulated in terms of exposure.
“We recognized in the laser bioeffects research that this rule that’s been around 20 or 30 years really was an error,” Sliney said. “It appeared that the statistics were showing that the hazard increased with many pulses. In fact, it does not.”
As a result, the allowable limits will be going up for repetitive pulses. Similarly, the limits for the shortest pulses, those that range from 100 fs to several picoseconds, are going up. Biophysics research is also behind a relaxation of the limits for the highest power pulses, according to Sliney.
Not every class of pulses will see a loosening of exposure limits. The allowable radiation exposure from single nanosecond-length pulses is going down, for example.
Industry-specific changes
There also are some changes that are related to specific products and industries. For instance, the movie industry is moving from xenon arc lamps to laser illumination. The way the standard was written, that transition required the use of ropes to keep moviegoers and others away, as well as a laser safety officer. The new light source, however, is no more dangerous than the old, according to the industry. Consequently, the new standard has specific provisions to eliminate the most burdensome laser safety requirements for projection applications.
As can be seen by such an example, setting the safety parameters for an embedded laser system is a complicated affair. Likewise, meeting the requirements can be a knotty challenge. It’s made more difficult because the safety systems add cost and complexity, which may make a laser-based solution less attractive than the alternatives.
Gus Anibarro, the education director of the Laser Institute of America, teaches the organization’s industrial laser safety officer (LSO) course and also a three-day LSO course. During this training, he goes over enclosures. The most popular in an industrial setting are made of steel; in research facilities, the enclosure is most often made of a combination of polycarbonate and brush, black anodized metal.
Containers can ensure no laser radiation escapes and so eliminate any chance of harm. Such an arrangement makes an otherwise dangerous laser harmless and allows it to be widespread.
A good example is a laser printer, Anibarro said. “Typically, these lasers are Class 3B. You can pull out the ink cartridge, pull out the paper bin, and you will never be exposed to the laser beam. This is a Class 1 laser system, and you probably have one in your office.”