New design and manufacturing techniques allow for challenging configurations of beamsplitters and diffractive axicon beam shapers.
SHLOMIT KATZ, NATAN KAPLAN AND ISRAEL GROSSINGER, HOLO/OR LTD.
Over the years, diffractive optics have evolved into an effective way of beam shaping and splitting. Their advantages over refractive optics are well known; they’re lightweight and compact and can be integrated easily into optical systems. They also can perform several optical functions in a single element.
Recent advances in diffractive optical elements (DOEs) have made them a standard component in laser material processing, medical and aesthetic lasers, and structured light projection systems.
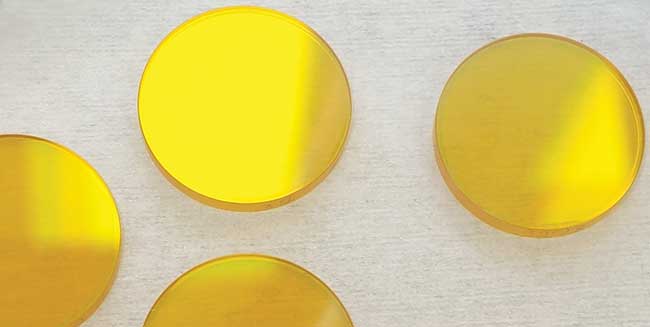
Diffractive optical elements made of zinc selenide glass. Courtesy of Holo/Or Ltd.
Enhancements in DOE design and manufacturing processes have reduced undesired orders and minimized zero order while improving uniformity and achieving higher diffractive efficiencies.
DOEs are diffractive microrelief patterns that manipulate the phase of an incoming laser beam in order to create a desired intensity profile at the far field (or lens focus-plane). As such, they are characterized by diffraction efficiency — the fraction of power that is directed into the desired direction versus the total input power. The fraction of power that is not in the desired pattern goes to higher orders at larger angles and to the zero order, which is a portion of the original beam that is not diffracted.
DOEs can be either a binary element — two-level design with typical efficiencies ~75 percent — or a multilevel element — four (or greater)-level design with efficiencies up to >95 percent. In general, the more levels used, the higher the efficiency and the lower the zero order. However, there are constraints that arise out of the manufacturing process.
The manufacturing process of a high-laser-damage-threshold DOE is based on photolithography and then dry plasma etching steps. Typical processed substrates include fused silica, zinc selenide and sapphire, all of which are ideal for high-power laser systems.
Manufacturing tolerances such as etching depth and feature size may induce high zero order and lower uniformity and, in general, degrade the overall performance of the DOE. For multilevel elements, these errors accumulate, and therefore manufacturing tolerances must be tighter.
To provide high-quality elements and reduce the unintentional lithography and etching errors, strict manufacturing tolerances are defined per design, taking into consideration the number of design levels and design specifications. DOEs can be divided into two broad categories: beam shaping and beam splitting.
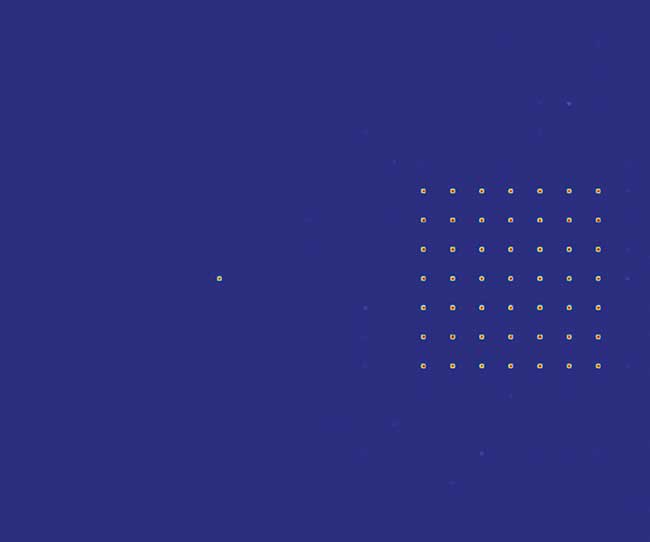
A 7 × 7 beamsplitter with zero order deflected from the main pattern. Courtesy of Holo/Or Ltd.
Beamsplitter DOEs are used to split a single laser beam into a predefined number of beams, each with the characteristics of the original beam and a desired angle of separation. A beamsplitter can generate either a one-dimensional beam array (1×N) or a two-dimensional beam matrix (M×N), depending on the diffractive pattern on the element. Beamsplitter specifications include diffractive efficiency, uniformity between the spots, zero-order value and undesired orders value.
Beam-shaper DOEs, on the other hand, are used to transform a Gaussian incident laser beam into a uniform-intensity spot of a round, rectangular, square, line or other shape with sharp edges in a specific work plane. Common products in this category are top hats, diffusers, diffractive axicons, vortex lenses and many more. Beam-shaper specifications include diffractive efficiency, transfer region and, in some cases, also zero order.
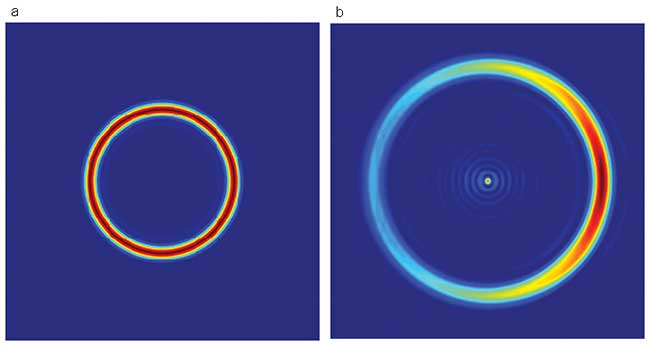
Comparison of typical behavior of binary axicon for misalignment (a) and typical behavior of a randomized array of diffractive axicons (RADA) for misalignment (b). Courtesy of Holo/Or Ltd.
Advances in DOE design and manufacturing techniques have led to improved designs for several “traditional” products, such as challenging configurations of beamsplitters and diffractive axicon beam shapers. In addition, new zero-order reduction methods are now possible, greatly improving the overall uniformity of the elements.
High-efficiency techniques
Double-spot (1 × 2 configuration) and quattro-spot (2 × 2 configuration) beam splitting are highly useful practices in many applications, including lithography, perforation, cutting and other materials processing applications. Although binary double-spot 1 × 2 beamsplitters and quattro-spot 2 × 2 splitters are highly robust and offer up to 81 percent and 64 percent efficiency, respectively, for demanding applications, the existence of even 1 percent input power in undesired higher orders is not tolerable.
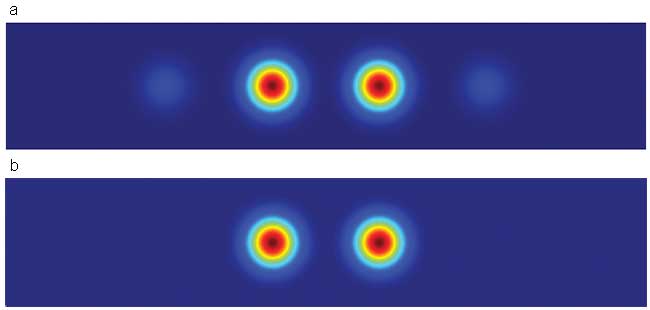
Comparison of a standard double-spot (a) and a high-efficiency double-spot (b). Courtesy of Holo/Or Ltd.

Comparison of a standard quattro-spot (a) and a high-efficiency quattro-spot (b). Courtesy of Holo/Or Ltd.
Recent advances in beamsplitter DOE techniques have led to improved designs with reduced undesired orders and higher diffractive efficiency of ~97 percent. These high-efficiency double-spot elements operate with a subaperture approach and are therefore sensitive to centering. The spot size in the splitting axis is increased and the spot becomes slightly elliptical, although this effect is felt only for single-mode lasers (low M2).
Spot size is defined by the formula shown below.

Equation 1
Where DLSM is the beam size for M2 = 1, EFL = effective focal length, λ = wavelength, D = input beam size, and M2 = the M2 value of the laser source; for low M2 (<~4), the beam in focus will have some ellipticity because of added 0.75, and for higher M2 values the spots will become symmetrical.
The high-efficiency quattro-spots increase the M2 value symmetrically; therefore no ellipticity will appear. However, spot size will increase up to ×1.4 for low M2 (single-mode) lasers.
Random array diffractive axicon
A diffractive axicon is a kind of DOE that transforms a laser beam into a ring shape (a Bessel intensity profile). An axicon also images a point source into a line along the optical axis and increases the depth of focus. Each diffractive axicon product is defined by its ring propagation angle. Typical applications for a diffractive axicon include laser corneal surgery, atomic traps, and Bessel-like beam generation.
Similar to other optical elements with axial symmetry, the diffractive axicon is sensitive to the centration of the DOE relative to the optical axis, but it is not sensitive to input beam size and M2 of the laser. Other mechanical tolerances have a minimal effect on functionality.
Following recent advances in DOE manufacturing, a new concept of designing a randomized array of diffractive axicons (RADA) was introduced. The RADA is designed to work with high-M2 lasers for applications such as laser heating of conductive surfaces (metals) or powder sintering, and is insensitive to centration alignment and beam symmetry. In addition, the multilevel design has a high diffractive efficiency of ~95 percent, as opposed to ~80 percent for standard binary design.
Zero-order reduction
As mentioned above, zero order is the fraction of the energy from the incident beam going through the DOEs without being “diffracted,” meaning the part of the incident beam obeying only the equations of geometrical ray optics (reflection and refraction).
Although in some cases the zero order is part of the designed image (array of spots), an issue remains with its energy variation compared to the design, especially in high-angle designs. Trying to bypass this issue can result in complex optical setups or abandoning the project altogether due to higher costs or insufficient performances. To overcome this challenge, engineers at Holo/Or Ltd. developed several techniques to suppress the zero order, each with its own fields of applicability.
Zero-order reduction by special diffractive design: As most DOEs are designed using an iterative Fourier transform algorithm, it is possible to give the zero-order optimization parameters larger weight in the overall merit function, over uniformity, efficiency, etc. This solution is the simplest one but has limited effectiveness, as it is only relevant for small-angle and small-number-of-spots beamsplitters.
Three phase-level diffractive structures instead of two: The introduction of a third phase level to the original binary grating profile can significantly reduce the design sensitivity to profile depth error (this translates into lower sensitivity to spectral width resulting in zero order). This solution is low cost (compared with eight- or 16-level designs), but it is useful only for multispectral diffuser applications.
Lateral shifting of the desired output image relative to zero order; adding a diffractive prism: The energy of the zero order (for example, 1 to 2 percent) continues on the optical axis, while the desired image is deflected in a certain angle according to the diffractive microprism array. This approach is less sensitive to misalignment compared to alternatives. The disadvantage is that the output image is laterally shifted compared to the optical axis, but this can be compensated by manufacturing on a wafer with a wedge. This design is also higher cost compared to a binary (two-level) DOE because of the addition of a multilevel microprism pattern and is limited to relatively small-angle applications.
Focal shifting of the desired output image relative to zero order; adding a diffractive (Fresnel) lens: By including a Fresnel type of lens, the possible energy of zero order spreads out and is evenly distributed over the entire region, appearing as a small background noise. Usually, the design is done so that the desired image will appear before the original focal plane; thus, the zero order will be focused at the focal plane. (It is also possible to move the zero order to another plane and leave the image plane at the original EFL of the system.) This on-axis technique can achieve higher efficiency compared to other solutions, but centration becomes more important. This solution is also higher cost compared to a binary DOE because of the addition of a multilayer Fresnel lens.
As DOEs became an effective and standard way of beam shaping and splitting in various industrial laser applications, some issues still needed to be resolved, such as the presence of undesired orders and high zero order. Recent enhancements in DOE design and manufacturing have made it possible to overcome these challenges and introduce improved designs with lower undesired orders, lower zero order, higher efficiency, higher uniformity and lower sensitivity to misalignment.
Further design improvements are being investigated constantly, along with different manufacturing techniques.
For many high-power laser applications such as materials processing, medicine and aesthetics, these enhancements allow higher precision and optimized performance.
Meet the authors
Shlomit Katz is an optical design engineer at Holo/Or Ltd. She designs diffractive optical elements and laser optical systems and provides technical support for a wide range of products. Katz holds a bachelor of science degree in optical systems engineering; email: [email protected].
Natan Kaplan is Holo/Or’s R&D manager. Specializing in subwavelength structures for antireflective purposes, he has more than 10 years of experience in micro-optics development, from both design and process sides. Kaplan has a master of science degree in applied physics; email: [email protected].
Israel Grossinger is founder and CEO of Holo/Or. Following an extensive career as HP-Indigo’s R&D manager, he founded Holo/Or in 1989, developed the diffractive optics technology and made it the leading company in this field. Grossinger holds a bachelor of science degree in electrical engineering; email:[email protected].
References
1. M. Duocastella and C. B. Arnold (2012). Bessel and annular beams for materials processing. Laser Photon Rev, Vol. 6, No. 5, pp. 607-621.v
2. A. Davis and F. Kühnlenz (December 2007). Optical design using Fresnel lenses. Optik & Photonik, Vol. 2, Issue 4, pp. 52-55.